Current state of the art imaging approaches for colorectal liver metastasis
Introduction
According to 2018 global cancer statistics, colorectal cancer (CRC) is the third most common cancer type and the second leading cause of cancer-related death among women and men (1). One of strongest predictors of long-term prognosis is the presence of synchronous metastasis at the time of diagnosis or developing metachronous metastasis during the disease course (2,3). The liver is the first and most common site of metastatic disease in the overwhelming majority of patients (2). The overall survival of patients with CRC has dramatically improved over the last several decades, especially in developed countries, given the implementation of screening programs to detect the primary tumor, as well as due to advances in therapeutic options for patients with colorectal liver metastases (CRLM) (1,4,5). While a variety of treatment options are available for synchronous or metachronous CRLM, surgical resection remains the cornerstone modality that is associated with the best chance for long-term survival (6,7). However, patients with CRLM represent a heterogeneous population with a wide variety of liver involvement and, therefore, not all patients are suitable candidates for hepatic resection (8). In fact, even after curative-intent resection, the incidence of recurrence can be as high as 30–50% following surgery depending on the number, size, and genetic profile of metastases; in addition, the volume of remaining healthy liver is also an important prognostic factor especially relative to peri-operative outcomes (9-11). Medical imaging plays a substantial role in defining the best therapeutic approach for CRLM patients, including the suitability of surgical management relative to other treatment modalities such as systemic or loco-regional therapies [e.g., neoadjuvant chemo-radiotherapy, radiofrequency ablation (RFA), transarterial chemoembolization (TACE)]. In this article we review the current state-of-art imaging approaches for the initial diagnosis and surveillance of patients with CRLM.
Staging
Following the diagnosis of any cancer, the determination of disease stage is important to help define an optimal treatment plan, as well as inform overall patient prognosis. Based on the latest guideline of the American Joint Committee on Cancer (AJCC), TNM system is one of the most accepted staging systems for CRC worldwide (12). In the TNM staging paradigm, T stands for tumor size and its local extension, N represents local lymph node extension and M represents metastasis of the tumor to distant sites. Based on TNM, CRC has been categorized to 5 stages ranging from stage 0 (very early stage of the disease) to stage 4 (distant sites metastasis such as liver). Imaging plays an important role in TNM staging specially in detecting metastatic lesions. Currently, different imaging modalities are available for detection of CRLM including computed tomography (CT), magnetic resonance imaging (MRI) and fluorine 18 fluorodeoxyglucose (FDG) positron emission tomography (PET). CT is the most commonly used imaging method at most centers, however MRI should be used when detecting tumors smaller than 1 cm in the liver, as well as among patients with hepatic steatosis (13). Furthermore, multimodality imaging can provide more comprehensive information about the disease than use of a single modality alone. For example, although CT and MRI are the preferred modalities in detecting extra-hepatic and hepatic metastasis, the combination of functional and anatomical imaging modalities such as FDG-PET/CT and/or FDG-PET/MRI can improve the diagnostic performance of detecting intrahepatic and extrahepatic colorectal cancer metastases (14).
CT
CT is the most commonly used imaging modality for the detection of colorectal metastasis (15,16). Multidetector computed tomography (MDCT) is preferred for staging as it can provide information about the chest, abdomen and pelvis in less time compared with other modalities (17). MDCT scanners with submillimeter detectors can scan the whole liver in a single breath hold with submillimeter collimation for more precise diagnosis. Axial slice thickness of 2–4 mm may be the best slice thickness for viewing liver metastatic lesions in practice (18).
Contrast-enhanced computed tomography (CE-CT) is the mainstay modality for staging patients with CRC (16). CRLM lesions are defined by their hypovascularity. CRLM typically appear as hypodense lesions in pre-contrast CT images, and as hypodense foci with a peripheral hyperdensity zone (rim-enhancement; i.e., target sign) in arterial phase images (19). Since liver colorectal metastases are hypovascular, the lesions can be best seen on portal venous phase images as hypoenhancing lesions in the background of enhancing liver parenchyma. The reported sensitivity of CE-CT for detecting liver metastases is within the range 52% to 84.6% (20). Pre-contrast phase images alone are limited in differentiating CRLM from non-enhancing lesions like hepatic cysts (21,22); arterial phase images are superior for detection of hypervascular hepatic lesions (e.g., neuroendocrine, hepatocellular) that are different from CRLM. CT has an acceptable precision for initial detection of CRLM lesions (19). However, CT has a limited performance for detecting CRLMs smaller than 1 cm (Figure 1) (23). Scanning at slice thickness of 1 mm has not been shown to improve detection of liver lesions (24). More recently, MRI has been increasingly utilized for detecting CRLM due to its better sensitivity than CE-CT (25).
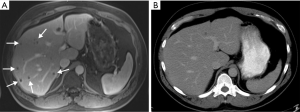
MRI
In general, CRLM lesions can be diagnosed by several characteristic MRI features. These features include T1 hypointensity, T2 hyperintensity, restricted diffusion in diffusion weighted imaging (DWI) MRI, and hypoenhancement in gadolinium-based contrast-enhanced MRI (CE-MRI) (Figure 2) (26,27). Typically, CRLM demonstrate peripheral rim enhancement on the arterial phase and appear hypointense in the portal venous phase with delayed phase of enhancement (28). Not all CRLMs have these typical features, however, and some may be missed by MRI.
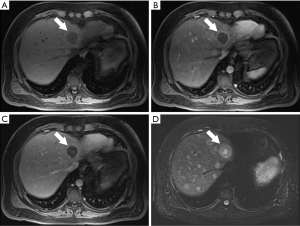
New MRI sequences such as DWI have improved accuracy in the detection and characterization of CRLM. DWI is based on measuring the Brownian motion of water molecules and uses the diffusion of water molecules to generate contrast on MRI images. One of the most important parameters of DWI-MRI is the apparent diffusion coefficient (ADC). The ADC map is a graphical display created by calculating the ratio of signal intensity in at least two DWI images with different weighting degrees (b value) (Figure 3). DWI has been shown to be more sensitive than T2-weighted MRI for detection of CRLM (29). In fact, DWI-MRI has a sensitivity of 82% and a specificity of 90% as an initial modality for detecting distant metastases (30). One major advantage of DWI is its use for detection of liver metastasis in patients with contraindication to contrast administration due to poor renal function or allergy, which is a common issue in CT and MRI with contrast imaging (31).
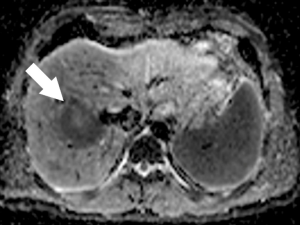
New hepatobiliary contrast agents have increased MRI sensitivity for detecting liver metastasis (32). Gadolinium-ethoxybenzyl diethylenetriamine pentaacetic acid (Gd-EOB-DTPA, marketed as Gadoxetic acid, or Eovist, Bayer, Germany) has 50% excretion via the biliary system and 50% excretion via glomerular filtration (33). This contrast agent allows dynamic imaging and shows different enhancement characteristics for various liver diseases. In the hepatobiliary phase (~20 min), Eovist is taken up selectively by functioning hepatocytes. Metastatic lesions do not take up hepatobiliary contrast and appear as hypointense lesions versus the brightly enhanced normal liver parenchyma (Figure 4) (34). Hepatobiliary contrast enhanced MRI is known to be the most sensitive imaging modality for detection of CRLM (35). Gd-EOB-DTPA-MRI has better performance to detect CRLM compared with ECM (extracellular matrix) CE-MRI or CE-MDCT (36). DWI-MRI has similar sensitivity and specificity versus ECM CE-MRI for the detection of CRLM lesions (37) but this modality has less sensitivity than Gd-EOB-DTPA-MRI (30). Combination of DWI with either ECM CE-MRI or Gd-EOB-DTPA-MRI has a better precision than DWI-MRI or ECM CE-MRI alone (30,37). Overall, the sensitivity of ECM CE-MRI for detection of CRLM is within a range 76% to 85.7% and is superior to sensitivity of other modalities such as CE-CT (38). This superiority is more obvious for small lesions (13). Of note, MRI has limited sensitivity for identifying extrahepatic metastatic lesion, which limits its role in CRC staging (19). Moreover, not all patients benefit from the high sensitivity of MRI for detection of CRLM. Like all other MRI studies, patients need to be screened for contraindications including metal devices and implants. In addition, lack of patient compliance may result in significant breathing and motion artifacts.
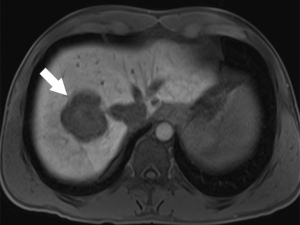
FDG-PET/CT
PET/CT can provide both anatomical and functional information about the tumors. The 18FDG used in PET/CT is a glucose analogue and can be traced to the tumor (Figure 5) (39). Standardized uptake value (SUV) is one of the important parameters for evaluating the metabolic uptake of 18FDG in tumor cells (39). SUV has better reproducibility than the other standardized measurable metrics (39). The major advantage of FDG PET/CT is its superiority in identifying extrahepatic metastatic lesions with a high accuracy up to 97%. FDG PET/CT is also useful to identify liver metastasis (Figure 5) (40-42). In one meta-analysis, the sensitivity and specificity of PET/CT for CRLM detection was reported to be 74.1% and 93.9%, respectively (43).
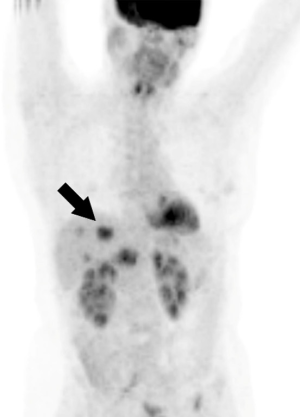
One limitation of PET that needs to be considered is that not all CRLMs are detectable by FDG. For example, mucinous tumors that account for approximately 17% of CRC tumors have no FDG uptake. Therefore, PET should not be utilized in this group of tumors (44). Moreover, PET/CT has a limited sensitivity in detecting metastatic tumors less than 10 mm.
FDG-PET/MRI
The role of FDG-PET combined with MRI has recently been introduced as a diagnostic method of detecting CRC metastasis. Kang et al. demonstrated the added value of PET-MRI to CT to detect extrahepatic CRC metastasis (45) while another study reported that PET-MRI can improve the limited sensitivity of PET-CT to detect small lesions (46). In general, the combination of PET and MRI can improve the detection of both intra- and extrahepatic lesions over PET/CT alone.
Staging in patients who have received chemotherapy
The use of chemotherapy among patients with CRC plays an important role in improving patient prognosis. Systemic chemotherapy can result, however, in changes to the normal liver parenchyma as well as tumor features, limiting the sensitivity of cross-sectional imaging to detect CRLM lesions in the liver (47,48). For example, irinotecan can cause steatosis in up to 20% of patients (47,49). In turn, liver steatosis can cause hyper-echogenicity of the liver parenchyma that can affect the performance of ultrasound for detection of the CRLM lesions (50,51). CT has less sensitivity for CRLM detection among patients who have received chemotherapy for the primary CRC or in the preoperative setting for CRLM (19). Rather, MRI has been demonstrated in a meta-analysis to be the best modality choice for the diagnosis of CRLM lesions after systemic chemotherapy (32).
Pre-surgical planning
Imaging modalities
MRI
For the differentiation between benign hepatic lesions and CRLM, DWI-MRI can be very helpful (37). ADC measurements tend to be higher in benign than metastatic lesions, although there is still overlap with some benign lesions (52,53). Gd-EOB-DTPA-MRI can identify equivocal small hepatic lesions on CT with high accuracy and also can detect additional lesions that were missed by CT (26). The cost of Gd-EOB-DTPA-MRI is higher compared with ECM CE-MRI or CE-MDCT. However, since Gd-EOB-DTPA-MRI has better performance, it can decrease overall cost of imaging. In particular, Gd-EOB-DTPA-MRI is increasingly being used as the initial imaging modality for detecting CRLM lesions before surgery planning (36).
PET/CT
There are several studies that have demonstrated that PET/CT can change pre-surgical decisions due to extrahepatic findings not detected on CE-CT (54,55). In a study on 65 patients with CRC, FDG PET/CT identified extrahepatic metastatic lesions in 17% of cases leading to a change in surgical management (54). The Royal College of Physicians have defined some of the clinical uses of PET/CT: staging of patients with resectable tumors, better diagnosis of equivocal tumors, as well as pretreatment restaging among patients with possible recurrence (55). Considering the additional value in the detection of extrahepatic metastasis, FDG PET/CT can be included in the pre-surgical evaluation of patients with colorectal cancer even when liver metastasis is known (19).
While there are several treatment methods available for patients with CRLM, surgery is the most effective treatment for improving survival. Ideally, resection of CRLM involves total tumor resection with microscopically negative margins while maintaining as much normal liver parenchyma as possible. Accurate imaging can greatly facilitate treatment planning prior to surgery.
CRLM prognostic features
The known prognostic factors for CRLM lesions include number of metastases, size and location of the lesions, response to systemic chemotherapy, hypo echogenicity of the tumor on ultrasound, presentation of disease (e.g., synchronous vs. metachronous), vascular invasion/proximity to vessels, as well as the quality of the underlying liver (56).
Liver parenchyma
Surgeons usually determine the surgical plan based on the technical resectability of the disease, which includes the need to leave an adequate amount of normal liver following resection (49). Generally, 25% to 30% of functional liver remnant (FLR) with appropriate inflow and outflow must be preserved in order for surgical resection to be possible without the risk of liver insufficiency or failure. In the setting of preoperative chemotherapy, diabetes, or a steatotic liver, the goal FLR should be more in the range of 30% or even 40% if there are signs of liver fibrosis (47). Measurement of the liver total volume can be done by CT or MRI, which are reported to have similar performance in calculating liver volumetry (57). Including vessels can result in an overestimation of about 13% in total liver (58). Liver volumetry needs more observation in patients who have undergone chemotherapy and have hepatic steatosis (59). Determining tumor burden can be possible after tumor volumetry (60). In addition to determining the FLR, anatomical variations within the liver parenchyma can have a role in determining resectability. CT and MRI are both good modality for pre-surgical planning, however MRI is better in defining biliary tract anatomy and CE-CT has better performance to indicate vasculature anatomy of the liver.
The CRLM tumors are often considered resectable, potentially resectable or non resectable. Potentially resectable tumors can become resectable after systemic chemotherapy or loco-regional therapies like preoperative portal vein embolization (PPVE) to decrease the size of the tumor or increase the liver segments’ volume, respectively (61). Tumor features and liver parenchyma can be evaluated again before extra chemotherapy side effects (59).
Extra hepatic involvement
Identification of extrahepatic tumors is also important for surgical decision-making because it can change the overall therapeutic plan. Typically, either CT alone of the chest and abdomen, or PET/CT is the preferred means to identify systemic disease. In particular, PET/CT has been reported to impact decision making in a subset of patients with CRLM whose disease may otherwise been missed on routine cross-sectional imaging (62).
Follow up
Assessing response to treatment is critical in defining the next steps in the treatment plan and estimating prognosis among CRC patients. Tumor response can be evaluated by the “Response Evaluation Criteria In Solid Tumor” (RECIST), which is a morphologic-based criteria. RECIST evaluates response by assessing the change in size of the tumor. These criteria do not, however, take into account information about the intra-lesional features (63). Recent studies have confirmed that functional criteria can evaluate tumor response earlier than anatomical criteria. As such, tumor response can be measured using 2D axial slice or by semi-automatic 3D segmentation techniques. The latter may provide additional data about the whole tumor with higher reproducibility compared with only measuring the region of interest (ROI) area with 2D images (15,64). The functional characterization of the tumor can assist in predicting response, recurrence of the disease and survival. Imaging is also essential in the follow-up of CRLM patients, and is typically done every 3 to 6 months for the first 3 years and then every 6 months for 3–5 years. CT and MRI are the most commonly used modalities for surveillance purposes (38).
Treatment methods
Early prediction of treatment response is very important because there are several treatment methods that can be used in non-responders. In addition to surgical resection, other treatments for CRLM include systemic chemotherapy, RFA, Microwave ablation, TACE, and selective internal radiation therapy (SIRT). RFA and microwave ablation can be alternatives for patients who are not proper candidates for surgery. Ablation destroys tumor cells by an image guided needle. Proper ablation can be achieved when both the whole tumor and as well as safe margin of the normal liver parenchyma are destroyed within the ablation region. Post-ablation results can be evaluated best by contrast enhanced CT or MRI during the portal vein phase. Unsuccessful ablation can result in residual tumor and disease progression (65,66). In fact, recurrence following ablation can be as high as 20% to 60% (67,68). Hence, patients should be monitored after ablation for early diagnosis of progression and recurrence by examining the margin of the ablation area (69).
Systemic chemotherapy is another important treatment option that is frequently used in the treatment of primary CRC and CRLM. Currently FOLFOX (including Leucovorin Calcium, Fluorouracil and Oxaliplatin) and FOLFIRI (including Leucovorin Calcium, Fluorouracil and Irinotecan Hydrochloride) are the most commonly used chemotherapy regimens for CRLM. These treatments can decrease the size, number and viability of the tumors (70). Bevacizumab is a monoclonal antibody drug that can be added to chemotherapy regimens to blocks angiogenesis within the tumor. Early response evaluation after systemic treatment can be accomplished using morphologic and functional imaging features to make decisions around treatment response, continued use of a certain chemotherapeutic agent, or change to alternative regimens or loco-regional treatment.
In addition to systemic chemotherapy, TACE and SIRT are two loco-regional treatments that have cytotoxic tumor effects. TACE is a procedure that involves embolization of the arterial supply of the hepatic lesion and can be combined with delivery of chemotherapeutic drugs locally. SIRT is another loco-regional treatment method in which radioembolization is used to treat the tumor. During this procedure, radioactive microspheres are released in the blood supply of the tumor. SIRT typically needs more time than TACE before there are detectable changes in the appearance of the tumor on functional imaging. Response to all these treatments can be evaluated by CT or MRI.
Systemic chemotherapy-related considerations
Sinusoidal obstruction syndrome (SOS) is a side effect of chemotherapy that can be associated with oxaliplatin in up to 20% of patients (39). CT can identify signs of SOS including hepato-splenomegaly and portal vein thrombosis (38,71). Oxaliplatin can also cause focal nodular hyperplasia like lesions. These lesions can have similar imaging features as FNH-like hyperintensity on the post contrast MRI images (72).
Systemic chemotherapy can also result in downsizing of liver tumors to the point that the lesions disappear—so called “disappearing liver metastasis” (DLM) lesions (73). It is important to note, however, that just because the lesion has disappeared on imaging does not necessarily mean that there has been a complete histopathological response. In fact, DLMs can be found in up to 80% of patients (74). MRI can detect some of the DLMs even when no lesion is visible on CT. Specifically, multiparametric MRI with several sequences can have more efficacy in diagnosing DLM (62). Gd-EOB-DTPA-MRI is known to be the most sensitive preoperative modality for detection of DLMs (35); specifically, Gd-EOB-DTPA-MRI alone has a sensitivity of 48% and a positive predictive value of 92% on detecting DLM lesions. Adding Contrast Enhanced intra operational ultrasound (CE-IOUS) can increase the sensitivity up to 99% and positive predictive value up to 98% (75).
Response and survival
CT
Higher mean attenuation value on the pre-treatment CT is associated with better treatment response and overall survival (15). There are also other features related to CT texture analysis that are being investigated to better define treatment response and survival. Some of these CT texture variables include entropy, uniformity, skewness, kurtosis, standard deviation (SD) and the ratio of the tumor features to the liver. Entropy is correlated with heterogeneity, while uniformity is related to homogeneity. Skewness refers to an asymmetry of the distribution of signal density, kurtosis is peakedness in pixel histogram and SD from the mean attenuation can be a variable of heterogeneity. Higher skewness and higher SD suggests increased heterogeneity within a tumor (15,76).
There is a discrepancy among recent studies regarding the prediction of treatment response and prognosis based on tumor heterogeneity and the portal venous phase of CE-CT. Some studies have not identified strong predictors and other reports have noted opposite results relative to the baseline heterogeneity (15,77,78). Some studies have reported that entropy in the liver is higher with an occult malignancy than a liver that has no metastatic disease (79-82). There is a pathological correlation between homogeneity and tumor grading that can be explained by dense cellularity of the tumor (77).
Post-treatment, CT responders have less entropy and higher uniformity. In contrast, among responders, most parts of the tumor become necrotic with more homogeneity whereas among non-responders some parts are still viable (83). Homogeneity features in post-chemotherapy CT has also been associated with better overall survival (84). Decrease in CT attenuation can predict early response after loco-regional treatments (85). Remaining viable parts in the treated tumor may be associated with less response to the treatment. If a tumor has residual rim enhancement on the post-treatment CE-CT, it may have viable parts in it; this finding has been confirmed by pathology correlation (86). There are, however, some limitations with CT imaging after cytotoxic treatments. As noted, post-chemotherapy liver steatosis causes lower attenuation in the image resulting in difficulties during the follow up which makes the MRI the better method to evaluate post treatment CRLM (65).
MRI
MRI has been proposed to be the best modality for assessing response and monitoring treatment effect after most of treatments (38). One of the most important features to be evaluated is rim enhancement, as the remaining viable portions of the tumor are mostly in the periphery of the lesions after chemotherapy (87-89). Lower pre-operative tumor enhancement is associated with tumor necrosis in post-operative pathological studies. Tumors with higher enhancement are known to have better biology and therefore better survival (90). Pre-treatment Gd-EOB-DTPA-MRI can predict response, as patients with stronger delayed enhancement will respond better to the treatments and live longer (91). Response is usually defined by RECIST and mRECIST criteria in the clinical setting.
DWI-MRI may be utilized to predict response to the cytotoxic treatments (92). Mean ADC before and after the treatment have a strong relation with the final change in size of the CRLM lesion (93). There are still some discrepancies among recent studies. However, in general, low pre-treatment mean ADC can better predict outcomes (94). High pretreatment ADC has been pathologically confirmed to be correlated with necrosis (95). Less perfusion in these regions causes them to receive less chemotherapy that, in turn, causes worse tumor response (31,93,96). There is, however, no known correlation between Intravoxel incoherent motion (IVIM) and the pathologic viable regions (95). ADC also has a relation with tumor proliferation degree that can determine the cell density in a tumor (93,97).
Post-treatment response can be evaluated by increases in mean ADC. Treatments can damage tumor cell membranes and cause necrosis and fibrosis. These changes cause the water molecules to move freely. Therefore parts of the tumor with necrosis have higher ADC than viable parts (Figure 6) (98-100). ADC can evaluate the response in less than 2 days after treatment (101). There is a correlation between ADC of the tumor in post-treatment response and pathologic studies. Non-responding tumors have a higher tumor regression grade and lower ADC than responders (102). Some studies have reported that ROI on the entire tumor did not correlate with the residual tumor, while ROI on the periphery can represent residual tumor; (89) there are other studies that have reported ADC on the entire tumor ROI, the tumor center, or on the periphery can effectively categorize the tumor response (102).
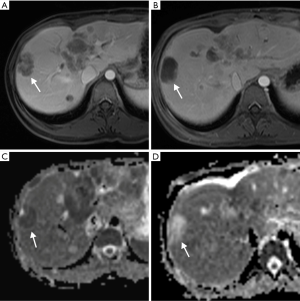
PET/CT
PET/CT can provide information about both the anatomical and functional features of the tumor with SUV being associated with the metabolic activity of the tumor. Glucose metabolism increases in cancer cells and is associated with more aggressive types of the tumor (39,76,103). Higher 18F-FDG uptake in a tumor represents more viable parts of the tumor (39). A higher pre-treatment SUV is associated with a more pathological viable tumor, which can be associated with prognosis even more than demonstration of tumor shrinkage post-treatment (104). In fact, tumors with higher SUV in the pre-treatment imaging have a worse overall survival due to the more aggressive nature of these tumors (39). These tumors also have more risk of metabolic progression (105). As such, a higher metabolic tumor volume is a strong predictor of worse survival (106). In the post-treatment setting, a decrease in SUV on PET/CT has been associated with a good response, and has been associated with a greater chance of the patient being a complete responder and having a better long-term outcome (105).
Conclusions
In conclusion, medical imaging plays an important role in CRLM management. The current review provides an overview of imaging approaches during staging, pre-treatment planning and follow up of patients with CRLM. CT is the mainstay of staging in most cancer centers and MRI has higher accuracy in primary diagnosis of synchronous and metachronous CRLM. PET-CT is a useful modality in detecting extra-hepatic metastatic lesions. Currently new predictive models of different imaging modalities before or early after treatment provide a better estimation of treatment response. Early prediction of response can assist in future planning of patient management and may avoid potential side effects of un-necessary treatment.
Acknowledgments
None.
Footnote
Conflicts of Interest: The authors have no conflicts of interest to declare.
Ethical Statement: The authors are accountable for all aspects of the work in ensuring that questions related to the accuracy or integrity of any part of the work are appropriately investigated and resolved.
References
- Bray F, Ferlay J, Soerjomataram I. Global Cancer Statistics 2018: GLOBOCAN Estimates of Incidence and Mortality Worldwide for 36 Cancers in 185 Countries. CA Cancer J Clin 2018;68:394-424. [Crossref] [PubMed]
- Okholm C, Mollerup TK, Schultz NA, et al. Synchronous and metachronous liver metastases in patients with colorectal cancer. Dan Med J 2018;65:A5524. [PubMed]
- Manfredi S, Lepage C, Hatem C, et al. Epidemiology and Management of Liver Metastases From Colorectal Cancer. Ann Surg 2006;244:254-9. [Crossref] [PubMed]
- Arnold M, Sierra MS, Laversanne M, et al. Global patterns and trends in colorectal cancer incidence and mortality. Gut 2017;66:683-91. [Crossref] [PubMed]
- Schreuders EH, Ruco A, Rabeneck L, et al. Colorectal cancer screening : a global overview of existing programmes. Gut 2015;64:1637-49. [Crossref] [PubMed]
- Viganò L, Ferrero A, Tesoriere R, Lo , et al. Liver Surgery for Colorectal Metastases: Results after 10 Years of Follow-Up. Long-Term Survivors, Late Recurrences, and Prognostic Role of Morbidity. Ann Surg Oncol 2008;15:2458-64. [Crossref] [PubMed]
- Alberts SR, Poston GJ. Treatment Advances in Liver-Limited Metastatic Colorectal Cancer. Clin Colorectal Cancer 2011;10:258-65. [Crossref] [PubMed]
- House MG, Ito H, Gönen M, et al. Survival after Hepatic Resection for Metastatic Colorectal Cancer: Trends in Outcomes for 1, 600 Patients during Two Decades at a Single Institution. J Am Coll Surg 2010;210:744-52. [Crossref] [PubMed]
- Littlechild J, Junejo M, Simons A, et al. Emergency resection surgery for colorectal cancer: Patterns of recurrent disease and survival. World J Gastrointest Pathophysiol 2018;9:8-17. [Crossref] [PubMed]
- Fong Y, Fortner J, Sun RL, et al. Clinical Score for Predicting Recurrence After Hepatic Resection for Metastatic Colorectal Cancer Analysis of 1001 Consecutive Cases. Ann Surg 1999;230:309-18. [Crossref] [PubMed]
- Jones RP, Jackson R, Dunne DFJ, et al. Systematic review and meta-analysis of follow-up after hepatectomy for colorectal liver metastases. Br J Surg 2012;99:477-86. [Crossref] [PubMed]
- Amin M, Edge S, Greene F, et al. AJCC Cancer Staging Manual. 8th ed. New York, NY Springer. 2017;
- Niekel MC, Bipat S, Stoker J. Diagnostic Imaging of Colorectal Liver Metastases with CT, MR Imaging, FDG PET, and/or FDG PET/CT: A Meta-Analysis of Prospective Studies Including Patients Who Have Not Previously Undergone Treatment. Radiology 2010;257:674-84. [Crossref] [PubMed]
- Brendle C, Schwenzer NF, Rempp H, et al. Assessment of metastatic colorectal cancer with hybrid imaging: comparison of reading performance using different combinations of anatomical and functional imaging techniques in PET/MRI and PET/CT in a short case series. Eur J Nucl Med Mol Imaging 2016;43:123-32. [Crossref] [PubMed]
- Ahn SJ, Kim JH, Park SJ, et al. Prediction of the therapeutic response after FOLFOX and FOLFIRI treatment for patients with liver metastasis from colorectal cancer using computerized CT texture analysis. Eur J Radiol 2016;85:1867-74. [Crossref] [PubMed]
- Fowler KJ, Kaur H, Cash BD, et al. ACR Appropriateness Criteria®Pretreatment Staging of Colorectal Cancer. J Am Coll Radiol 2017;14:S234-44. [Crossref] [PubMed]
- Schima W, Kulinna C, Langenberger H, et al. Liver metastases of colorectal cancer: US, CT or MR? Cancer Imaging 2005;5 Spec No A:S149-56.
- Mainenti PP. Non-invasive diagnostic imaging of colorectal liver metastases. World J Radiol 2015;7:157-69. [Crossref] [PubMed]
- Rojas Llimpe FL, Di Fabio F, Ercolani G, et al. Imaging in resectable colorectal liver metastasis patients with or without preoperative chemotherapy: Results of the PROMETEO-01 study. Br J Cancer 2014;111:667-73. [Crossref] [PubMed]
- Vreugdenburg TD, Ma N, Duncan JK, et al. Comparative diagnostic accuracy of hepatocyte-specific gadoxetic acid (Gd-EOB-DTPA) enhanced MR imaging and contrast enhanced CT for the detection of liver metastases : a systematic review and meta-analysis. Int J Colorectal Dis 2016;31:1739-49. [Crossref] [PubMed]
- Valls C, Andía E, Sánchez A, et al. Hepatic Metastases from Colorectal Cancer: Preoperative Detection and Assessment of Resectability with Helical CT. Radiology 2001;218:55-60. [Crossref] [PubMed]
- Ch’en IY, Katz DS, Jeffrey RB, et al. Do arterial phase helical CT images improve detection or characterization of colorectal liver metastases? J Comput Assist Tomogr 1997;21:391-7. [Crossref] [PubMed]
- Tirumani SH, Won Kim K, Nishino M, et al. Update on the Role of Imaging in Management of Metastatic Colorectal Cancer 1. RadioGraphics 2014;34:1908-28. [Crossref] [PubMed]
- Kulinna C, Helmberger T, Kessler M, et al. Improvement in diagnosis of liver metastases with the multi-detector CT. Radiologe 2001;41:16-23. [Crossref] [PubMed]
- Sivesgaard K, Larsen LP, Sørensen M, et al. Diagnostic accuracy of CE-CT, MRI and FDG PET/CT for detecting colorectal cancer liver metastases in patients considered eligible for hepatic resection and/or local ablation. Eur Radiol 2018;28:4735-47. [Crossref] [PubMed]
- Cho JY, Lee YJ, Han HS, et al. Role of gadoxetic acid-enhanced magnetic resonance imaging in the preoperative evaluation of small hepatic lesions in patients with colorectal cancer. World J Surg 2015;39:1161-6. [Crossref] [PubMed]
- Namasivayam S, Martin DR, Saini S. Imaging of liver metastases: MRI. Cancer Imaging 2007;7:2-9. [Crossref] [PubMed]
- Hamm B, Thoeni RF, Gould RG, et al. Focal liver lesions: characterization with nonenhanced and dynamic contrast material-enhanced MR imaging. Radiology 1994;190:417-23. [Crossref] [PubMed]
- Coenegrachts K, Delanote J, Ter Beek L, et al. Improved focal liver lesion detection: comparison of single-shot diffusion-weighted echoplanar and single-shot T 2 weighted turbo spin echo techniques. Br J Radiol 2007;80:524-31. [Crossref] [PubMed]
- Vilgrain V, Esvan M, Ronot M, et al. A meta-analysis of diffusion-weighted and gadoxetic acid-enhanced MR imaging for the detection of liver metastases. Eur Radiol 2016;26:4595-615. [Crossref] [PubMed]
- Scurr ED, Collins D, Temple L, et al. Appearances of colorectal hepatic metastases at diffusion-weighted MRI compared with histopathology: initial observations. Br J Radiol 2012;85:225-30. [Crossref] [PubMed]
- van Kessel CS, Buckens C, Van Den Bosch MAAJ, et al. Preoperative imaging of colorectal liver metastases after neoadjuvant chemotherapy: A meta-analysis. Ann Surg Oncol 2012;19:2805-13. [Crossref] [PubMed]
- Frydrychowicz A, Lubner M, Brown J, et al. Hepatobiliary MR Imaging with Gadolinium Based Contrast Agents. J Magn Reson Imaging 2012;35:492-511. [Crossref] [PubMed]
- Zech CJ, Herrmann K, Reiser M, et al. MR imaging in patients with suspected liver metastases: value of liver-specific contrast agent Gd-EOB-DTPA. Magn Reson Med Sci 2007;6:43-52. [Crossref] [PubMed]
- Owen JW, Fowler K, Doyle M, et al. Colorectal liver metastases: disappearing lesions in the era of Eovist hepatobiliary magnetic resonance imaging. HPB 2016;18:296-303. [Crossref] [PubMed]
- Zech CJ, Justo N, Lang A, et al. Cost evaluation of gadoxetic acid-enhanced magnetic resonance imaging in the diagnosis of colorectal-cancer metastasis in the liver: Results from the VALUE Trial. Eur Radiol 2016;26:4121-30. [Crossref] [PubMed]
- Barral M, Eveno C, Hoeffel C, et al. Diffusion-weighted magnetic resonance imaging in colorectal cancer. J Visc Surg 2016;153:361-9. [Crossref] [PubMed]
- Fowler KJ, Linehan DC, Menias CO. Colorectal liver metastases: State of the art imaging. Ann Surg Oncol 2013;20:1185-93. [Crossref] [PubMed]
- Xia Q, Liu J, Wu C, et al. Prognostic significance of18FDG PET/CT in colorectal cancer patients with liver metastases: A meta-analysis. Cancer Imaging 2015;15:19. [Crossref] [PubMed]
- Cho YB, Chun HK, Kim MJ, et al. Accuracy of MRI and 18 F-FDG PET/CT for Restaging After Preoperative Concurrent Chemoradiotherapy for Rectal Cancer. World J Surg 2009;33:2688-94. [Crossref] [PubMed]
- Maas M, Rutten I, Nelemans P, et al. What is the most accurate whole-body imaging modality for assessment of local and distant recurrent disease in colorectal cancer ? A meta-analysis Imaging for recurrent colorectal cancer. Eur J Nucl Med Mol Imaging 2011;38:1560-71. [Crossref] [PubMed]
- Moulton CA, Gu CS, Law CH, et al. Effect of PET before liver resection on surgical management for colorectal adenocarcinoma metastases a randomized clinical trial. JAMA 2014;311:1863-9. [Crossref] [PubMed]
- Choi SH, Kim SY, Park SH, et al. Diagnostic performance of CT, gadoxetate disodium-enhanced MRI, and PET/CT for the diagnosis of colorectal liver metastasis: Systematic review and meta-analysis. J Magn Reson Imaging 2018;47:1237-50. [Crossref] [PubMed]
- Grassetto G, Capirci C, Marzola MC, et al. Colorectal cancer: prognostic role of 18 F-FDG-PET / CT. Abdom Imaging 2012;37:575-9. [Crossref] [PubMed]
- Kang B, Lee JM, Song YS, et al. Added Value of Integrated Whole-Body PET/MRI for Evaluation of Colorectal Cancer: Comparison With Contrast-Enhanced MDCT. AJR Am J Roentgenol 2016;206:W10-20. [Crossref] [PubMed]
- Fraum TJ, Fowler KJ, Mcconathy J, et al. PET / MRI for the body imager: abdominal and pelvic oncologic applications. Abdom Imaging 2015;40:1387-404. [Crossref] [PubMed]
- Vauthey JN, Pawlik TM, Ribero D, et al. Chemotherapy Regimen Predicts Steatohepatitis and an Increase in 90-Day Mortality After Surgery for Hepatic Colorectal Metastases. J Clin Oncol 2006;24:2065-72. [Crossref] [PubMed]
- Pawlik TM, Olino K, Gleisner AL, et al. Preoperative Chemotherapy for Colorectal Liver Metastases : Impact on Hepatic Histology and Postoperative Outcome. J Gastrointest Surg 2007;11:860-8. [Crossref] [PubMed]
- Folprecht G. Liver Metastases in Colorectal Cancer. Am Soc Clin Oncol Educ 2018;book 36:e186-92.
- Catalano O, Nunziata A, Lobianco R, et al. Real-Time Harmonic Contrast Material– specific US ofFocal Liver Lesions. RadioGraphics 2005;25:333-49. [Crossref] [PubMed]
- van Vledder MG, Torbenson MS, Pawlik TM, et al. The Effect of Steatosis on Echogenicity of Colorectal Liver Metastases on Intraoperative Ultrasonography. Arch Surg 2010;145:661-7. [Crossref] [PubMed]
- Koh DM, Collins DJ. Diffusion-weighted MRI in the body: Applications and challenges in oncology. AJR Am J Roentgenol 2007;188:1622-35. [Crossref] [PubMed]
- Soyer P, Corno L, Boudiaf M, et al. Differentiation between cavernous hemangiomas and untreated malignant neoplasms of the liver with free-breathing diffusion-weighted MR imaging: Comparison with T2-weighted fast spin-echo MR imaging. Eur J Radiol 2011;80:316-24. [Crossref] [PubMed]
- Kong G, Jackson C, Koh DM, et al. The use of 18F-FDG PET/CT in colorectal liver metastases—comparison with CT and liver MRI. Eur J Nucl Med Mol Imaging 2008;35:1323-9. [Crossref] [PubMed]
- The Royal College of radialogists. Evidence-based indications for the use of PET-CT in the United Kingdom 2016. Clin Radiol 2016;71:e171-88. [Crossref] [PubMed]
- Spelt L, Andersson B, Nilsson J, et al. Prognostic models for outcome following liver resection for colorectal cancer metastases: A systematic review. Eur J Surg Oncol 2012;38:16-24. [Crossref] [PubMed]
- Pawlik TM, Scoggins C, Zorzi D, et al. Effect of Surgical Margin Status on Survival and Site of Recurrence After Hepatic Resection for Colorectal Metastases. Ann Surg 2005;241:715-22. [Crossref] [PubMed]
- Are C, Gonen M, Zazzali K, et al. The Impact of Margins on Outcome After Hepatic Resection for Colorectal Metastasis. Ann Surg 2007;246:295-300. [Crossref] [PubMed]
- Coimbra FJF, Ribeiro HS de C. First Brazilian Consensus on Multimodal Treatment of Colorectal Liver Metastases. Module 1: Pre-Treatment Evaluation. Arq Bras Cir Dig 2015;28:222-30. [Crossref] [PubMed]
- Misiakos EP, Karidis N, Kouraklis G. Current treatment for colorectal liver metastases. World J Gastroenterol 2011;17:4067-75. [Crossref] [PubMed]
- Abulkhir A, Limongelli P, Healey AJ, et al. Preoperative Portal Vein Embolization for Major Liver Resection. Ann Surg 2008;247:49-57. [Crossref] [PubMed]
- Fowler KJ, Linehan DC, Menias CO. Comment: Fancier and More Expensive Do Mean Better: An Argument for Computed Tomography Staging of Patients With Colorectal Liver Metastases. Ann Surg Oncol 2017;24:605-6. [Crossref] [PubMed]
- Eisenhauer EA, Therasse P, Bogaerts J, et al. New response evaluation criteria in solid tumours : Revised RECIST guideline (version 1. 1). Eur J Cancer 2009;45:228-47. [Crossref] [PubMed]
- Rothe JH, Grieser C, Lehmkuhl L, et al. Size determination and response assessment of liver metastases with computed tomography — Comparison of RECIST and volumetric algorithms. Eur J Radiol 2013;82:1831-9. [Crossref] [PubMed]
- Reiter MJ, Hannemann NP, Schwope RB, et al. Role of imaging for patients with colorectal hepatic metastases: what the radiologist needs to know. Abdom Imaging 2015;40:3029-42. [Crossref] [PubMed]
- Minami Y, Kudo M. Radiofrequency Ablation of Liver Metastases from Colorectal Cancer : A Literature Review. Gut Liver 2013;7:1-6. [Crossref] [PubMed]
- Samim M, Prevoo W, de Wit-van der Veen BJ, et al. 18F-FDG PET as novel imaging biomarker for disease progression after ablation therapy in colorectal liver metastases. Eur J Nucl Med Mol Imaging 2017;44:1165-75. [Crossref] [PubMed]
- Wong SL, Mangu PB, Choti MA, et al. American Society of Clinical Oncology 2009 Clinical Evidence Review on Radiofrequency Ablation of Hepatic Metastases From Colorectal Cancer. J Clin Oncol 2010;28:493-508. [Crossref] [PubMed]
- Ahmed M, Solbiati L, Brace CL, et al. Image-guided Tumor Ablation: Standardization of Terminology and Reporting Criteria—A 10-Year Update. Radiology 2014;273:241-60. [Crossref] [PubMed]
- Mansour MG, Ali SA. Transarterial chemoembolization using drug eluting microspheres in refractory colorectal liver metastases with 18F-FDG PET/CT follow-up to assess therapeutic response. Egypt J Radiol Nucl Med 2016;47:1467-72. [Crossref]
- Cayet S, Pasco J, Dujardin F, et al. Diagnostic performance of contrast-enhanced CT-scan in sinusoidal obstruction syndrome induced by chemotherapy of colorectal liver metastases: Radio-pathological correlation. Eur J Radiol 2017;94:180-90. [Crossref] [PubMed]
- Ünal E, Karaosmanoğlu AD, Ozmen MN, et al. Hepatobiliary phase liver MR imaging findings after Oxaliplatin-based chemotherapy in cancer patients. Abdom Radiol (NY) 2018;43:2321-8. [Crossref] [PubMed]
- Kingham TP, Pak LM, Simpson AL, et al. 3D image guidance assisted identification of colorectal cancer liver metastases not seen on intraoperative ultrasound: results from a prospective trial. HPB (Oxford) 2018;20:260-7. [Crossref] [PubMed]
- Benoist S, Brouquet A, Penna C, et al. Complete Response of Colorectal Liver Metastases After Chemotherapy: Does It Mean Cure ? J Clin Oncol 2006;24:3939-45. [Crossref] [PubMed]
- Arita J, Ono Y, Takahashi M, et al. Routine preoperative liver-specific magnetic resonance imaging does not exclude the necessity of contrast-enhanced intraoperative ultrasound in hepatic resection for colorectal liver metastasis. Ann Surg 2015;262:1086-91. [Crossref] [PubMed]
- Miles KA, Ganeshan B, Hayball MP. CT texture analysis using the filtration-histogram method: what do the measurements mean? Cancer Imaging 2013;13:400-6. [Crossref] [PubMed]
- Lubner MG, Stabo N, Lubner SJ, et al. CT textural analysis of hepatic metastatic colorectal cancer: pre-treatment tumor heterogeneity correlates with pathology and clinical outcomes. Abdom Imaging 2015;40:2331-7. [Crossref] [PubMed]
- Froelich MF, Heinemann V, Sommer W, et al. CT attenuation of liver metastases before targeted therapy is a prognostic factor of overall survival in colorectal cancer patients. Results from the randomised, open-label FIRE-3/AIO KRK0306 trial. Eur Radiol 2018;28:5284-92. [Crossref] [PubMed]
- Beckers RCJ, Lambregts DMJ, Schnerr RS, et al. Whole liver CT texture analysis to predict the development of colorectal liver metastases—A multicentre study. Eur J Radiol 2017;92:64-71. [Crossref] [PubMed]
- Rao SX, Lambregts DMJ, Schnerr RS, et al. Whole-liver CT texture analysis in colorectal cancer: Does the presence of liver metastases affect the texture of the remaining liver? United European Gastroenterol J 2014;2:530-8. [Crossref] [PubMed]
- Ganeshan B, Miles KA, Young RCD, et al. Texture analysis in non-contrast enhanced CT: Impact of malignancy on texture in apparently disease-free areas of the liver. Eur J Radiol 2009;70:101-10. [Crossref] [PubMed]
- Beckers RCJ, Trebeschi S, Maas M, et al. CT texture analysis in colorectal liver metastases and the surrounding liver parenchyma and its potential as an imaging biomarker of disease aggressiveness, response and survival. Eur J Radiol 2018;102:15-21. [Crossref] [PubMed]
- Rao SX, Lambregts DMJ, Schnerr RS, et al. CT texture analysis in colorectal liver metastases: A better way than size and volume measurements to assess response to chemotherapy? United European Gastroenterol J 2016;4:257-63. [Crossref] [PubMed]
- Chun YS, Vauthey J, Boonsirikamchai P. Association of computed tomography morphological criteria with pathologic response and survival in patients treated with bevacizumab for colorectal liver metastases. JAMA 2009;302:2338-44. [Crossref] [PubMed]
- Tochetto SM, Gurkan Tore H, Chalian H, et al. Colorectal Liver Metastasis After 90Y Radioembolization Therapy: Pilot Study of Change in MDCT Attenuation as a Surrogate Marker for Future FDG PET Response. AJR Am J Roentgenol 2012;198:1093-9. [Crossref] [PubMed]
- Ishida K, Tamura A, Kato K, et al. Correlation between CT morphologic appearance and histologic findings in colorectal liver metastasis after preoperative chemotherapy. Abdom Radiol (NY) 2018;43:2991-3000. [Crossref] [PubMed]
- Ng JKS, Urbanski SJ, Mangat N, et al. Colorectal Liver Metastases Contract Centripetally With a Response to Chemotherapy. Cancer 2008;112:362-71. [Crossref] [PubMed]
- Mentha G, Terraz S, Morel P, et al. Dangerous halo after neoadjuvant chemotherapy and two-step hepatectomy for colorectal liver metastases. Br J Surg 2009;96:95-103. [Crossref] [PubMed]
- Wagner M, Ronot M, Doblas S, et al. Assessment of the residual tumour of colorectal liver metastases after chemotherapy: diffusion-weighted MR magnetic resonance imaging in the peripheral and entire tumour. Eur Radiol 2016;26:206-15. [Crossref] [PubMed]
- Cheung HMC, Karanicolas PJ, Hsieh E, et al. Late gadolinium enhancement of colorectal liver metastases post-chemotherapy is associated with tumour fibrosis and overall survival post-hepatectomy. Eur Radiol 2018;28:3505-12. [Crossref] [PubMed]
- Cheung HMC, Karanicolas PJ, Coburn N, et al. Delayed tumour enhancement on gadoxetate-enhanced MRI is associated with overall survival in patients with colorectal liver metastases. Eur Radiol 2019;29:1032-8. [Crossref] [PubMed]
- Beckers RCJ, Lambregts DMJ, Lahaye MJ, et al. Advanced imaging to predict response to chemotherapy in colorectal liver metastases – a systematic review. HPB 2018;20:120-7. [Crossref] [PubMed]
- Cui Y, Zhang XP, Sun YS, et al. Apparent Diffusion Coefficient: Potential Imaging Biomarker for Prediction and Early Detection of Response to Chemotherapy in Hepatic Metastases. Radiology 2008;248:894-900. [Crossref] [PubMed]
- Liang HY, Huang YQ, Ding Y, et al. Potential of MR histogram analyses for prediction of response to chemotherapy in patients with colorectal hepatic metastases. Eur Radiol 2016;26:2009-18. [Crossref] [PubMed]
- Chiaradia M, Baranes L, Van Nhieu JT, et al. Intravoxel incoherent motion (IVIM) MR imaging of colorectal liver metastases: Are we only looking at tumor necrosis? J Magn Reson Imaging 2014;39:317-25. [Crossref] [PubMed]
- Tam HH, Collins DJ, Brown G, et al. The role of pre-treatment diffusion-weighted MRI in predicting long-term outcome of colorectal liver metastasis. Br J Radiol 2013;86:20130281. [Crossref] [PubMed]
- Gonzalez-Guindalini FD, Botelho M, Harmath C, et al. Assessment of Liver Tumor Response to Therapy: Role of Quantitative Imaging. Radiographics 2013;33:1781-800. [Crossref] [PubMed]
- Wagner M, Doblas S, Daire J, et al. Diffusion-weighted MR Imaging for the Regional Characterization of Liver Tumors. Radiology 2012;264:464-72. [Crossref] [PubMed]
- Padhani AR, Liu G, Mu-koh D, et al. Diffusion-Weighted Magnetic Resonance Imaging as a Cancer Biomarker: Consensus and Recommendations. Neoplasia 2009;11:102-25. [Crossref] [PubMed]
- Koh DM, Scurr E, Collins D, et al. Predicting response of colorectal hepatic metastasis: Value of pretreatment apparent diffusion coefficients. AJR Am J Roentgenol 2007;188:1001-8. [Crossref] [PubMed]
- Dudeck O, Zeile M, Wybranski C, et al. Early prediction of anticancer effects with diffusion-weighted MR imaging in patients with colorectal liver metastases following selective internal radiotherapy. Eur Radiol 2010;20:2699-706. [Crossref] [PubMed]
- Donati F, Boraschi P, Pacciardi F, et al. 3T diffusion-weighted MRI in the response assessment of colorectal liver metastases after chemotherapy: Correlation between ADC value and histological tumour regression grading. Eur J Radiol 2017;91:57-65. [Crossref] [PubMed]
- de Geus-Oei LF, Van Laarhoven HWM, Visser EP, et al. Chemotherapy response evaluation with FDG – PET in patients with colorectal cancer. Ann Oncol 2008;19:348-52. [Crossref] [PubMed]
- Nishioka Y, Yoshioka R, Gonoi W, et al. Fluorine-18-fluorodeoxyglucose positron emission tomography as an objective substitute for CT morphologic response criteria in patients undergoing chemotherapy for colorectal liver metastases. Abdom Radiol (NY) 2018;43:1152-8. [Crossref] [PubMed]
- Chiu KW, Lam KO, An H, et al. Long-term outcomes and recurrence pattern of 18F-FDG PET-CT complete metabolic response in the first-line treatment of metastatic colorectal cancer: A lesion-based and patient-based analysis. BMC Cancer 2018;18:776. [Crossref] [PubMed]
- Huang YTT, Park J, Chong S, et al. The prognostic value of fluorodeoxyglucose positron emission tomography metabolic tumor volume in solitary colorectal liver metastasis. Asia Pac J Clin Oncol 2017;13:e262-70. [Crossref] [PubMed]