Cytokinome of adult-derived human liver stem/progenitor cells: immunological and inflammatory features
Introduction
As the body’s major metabolic factory, the liver may be affected by different diseases or injury that could, in time, induce the loss of several vital functions. At present, liver transplantation is indicated as the best treatment for acute liver failure, congenital defects or end-stage cirrhosis, but many limitations hamper its efficiency (1). It is currently considered the gold standard treatment for end-stage liver disease, acute liver failure and liver-based metabolic disorders and is the only intervention with proven clinical benefits and long-lasting effects (2). Cell therapy has therefore emerged as an interesting therapeutic for a variety of liver diseases and for improving the success of liver transplantation (3). Hepatocytes were initially used for this therapy, but their clinical effects were moderate and short-lived. These cells are generally derived from livers inappropriate for transplant, limited in number, variable in quality, and not able to be expanded in vitro (4). Although clinical trials using various types of autologous cells have been performed to promote liver regeneration or reduce liver fibrosis, clear evidence of their therapeutic benefits is lacking. Cell types that have shown efficacy in preclinical models include hepatocytes, liver sinusoidal endothelial cells, mesenchymal stem cells, endothelial progenitor cells, and macrophages (5). Different cell sources (cell lines, stem cells, progenitor cells, and other non-parenchymal cell types) have therefore been proposed as alternative therapeutic liver cell products (4). By expressing both hepatic and mesenchymal markers, liver mesenchymal-like cells have been reported as a potential reservoir of hepatocytes (6-10). When exposed to in vitro hepatogenic differentiation (HD), adult-derived human liver stem/progenitor cells (ADHLSCs) may differentiate into hepatocyte-like cells, thus acquiring liver metabolic functions (11). They are also capable of inhibiting hepatic stellate cell (HSC) activation (12) and therefore contribute to liver regeneration by reducing local inflammation. Compared to other liver-derived cells, ADHLSCs have shown significantly distinct gene expression and secretion profiles (13). Accumulated experimental evidence supports the involvement of ADHLSCs in liver regeneration (14,15). However, some impairment in the engraftment process of ADHLSCs into the recipient liver has been observed. Extensive surface molecule screening has shown that ADHLSCs harbor differentially expressed molecules with potential implications for cell engraftment (16). Moreover, ADHLSCs are non-immunogenic and can suppress the lymphocyte response (17). Altogether, these findings indicate that ADHLSCs are an attractive alternative for cell-based liver therapies (5). Although ADHLSCs are still under investigation, they have been successfully used in the clinic due to their liver regeneration potential (18-20). Thus, infusing ADHLSCs following hepatectomy improved cell engraftment and hepatic tissue regeneration (21). Intrahepatic transplantation of ADHLSCs also significantly reduced liver fibrosis by inhibiting activated HSCs (22). Altogether, these findings confirm their potential as a liver therapeutic cell strategy, but their efficiency and safety must be addressed before widespread clinical application (23). Several concerns, such as the immune biology of the transplanted product and the response of the host, may critically influence the efficacy of the therapy (5,24). In vivo interactions between the cell graft and the host immune system are mediated by functional environmental sensors and stressors that play significant roles in the immunobiology of the graft (25). Accordingly, MSCs have also demonstrated marked plasticity regarding their phenotype and immunoregulatory effects that are dependent on the inflammatory environment. By balancing their pro- and anti-inflammatory fates, MSCs may centrally regulate the immune response during tissue repair and regeneration (26). Inflammation is thus a characteristic feature of several liver diseases and may differentially balance regeneration and fibrosis during liver repair (27). For instance, the immunological and inflammatory features of ADHLSCs are not clear. Both HD and stimulation with a mixture of inflammatory cytokines in vitro (I) influence the immunophenotype of ADHLSCs, with CD200 being the most altered immune marker (28). In parallel, inflammation was shown to significantly modulate several immunological cytokines within HSCs (29). However, the cytokinome of ADHLSCs after HD and after exposure to inflammatory cytokines has not been evaluated. By establishing the cytokinome of ADHLSCs and, most importantly, understanding their immunological and inflammatory features, we may provide some breakthroughs in the immunobiology of these cells, thus improving their therapeutic value for managing liver-associated diseases. In this study, we analyzed the cytokinome of ADHLSCs during HD and following stimulation with inflammatory cytokines in comparison to mature hepatocytes (the gold standard in liver transplantation) and demonstrated significantly different expression profiles for the major set of tested cytokines. Of note, these hepatocytes also responded to inflammatory priming by increasing the expression levels of most cytokines. Inflammation, rather than hepatic differentiation, may finely modulate the immunological balance of ADHLSCs.
Methods
Isolation and culture strategies of ADHLSCs and hepatocytes
All experiments were applied after the approbation of ethics committee of the Jules Bordet Institute and Université Libre de Bruxelles (ULB) and ethics committees of the St-Luc Hospital and faculty of Medicine of the Université Catholique de Louvain (UCL). Concerning the Hepatocytes and Hepatic Stem Cells Bank, an agreement from the Belgian Federal Agency for Medicines and Health Products was obtained. Finally, written and signed informed consent was obtained for all human liver used in this work. The isolation of hepatocytes and ADHLSCs were applied as previously described (8,20). In brief, human liver cells were obtained from the left liver segment originating from healthy cadaveric donors and cells isolated between 6 and 12 hours by a two-step collagenase perfusion technique in Hepatocytes and Hepatic Stem Cells tissue bank clean rooms (Laboratory of Pedriatic Hepatology and Cell Therapy, UCL). Following filtration and low-speed centrifugation, the parenchymal fraction (mostly compound by hepatocytes) was recovered and seeded as primary cultures. As previously described (3,20), the cells were cultured at 37 °C in a fully humidified atmosphere (5% CO2). Dulbecco’s modified Eagle’s medium (DMEM) with 4.5 g/L glucose (Life Technologies) supplemented with 10% fetal calf serum (FCS) (Life Technologies) and 1% penicillin/streptomycin (Life Technologies) was used to culture the cells. The cells were recovered (at 80% confluence) thanks to 0.05% Trypsin-EDTA (Life Technologies) and seeded on collagen type 1 coated plates at a density of 5,000 cells/cm2. The cells were finally collected at passage 6. This is after the second passage that the ADHLSCs become predominant [as previously shown (3)]. The purity of the culture of ADHLSCs was confirmed at the third passage by flow cytometry. This analysis aims to checking the immunopositivity for CD73, CD90, Alpha-smooth muscle actin, and immune-negativity for CD45. Immunocytochemistry and PCR are also used to demonstrate the expression of both mesenchymal (like ASMA, vimentin) and hepatocytic (like albumin) markers (data not shown).
HD of ADHLSCs
ADHLSCs were seeded in six-well plates coated with rat tail collagen type I (at a density of 104 cells/cm2) and cultures were maintained in expansion conditions for 48 h. After reaching 90% confluence, ADHLSCs were sequentially incubated with HD cocktail containing specific growth factors and cytokines in Iscove’s Modified Dulbecco’s Medium (IMDM; Life Technologies) as previously described (6). For the step 1 [20 ng/mL epidermal-growth factor (EGF) and 10 ng/mL basic fibroblast-growth factor (bFGF)], which lasted for 2 days, only one medium change was performed. For step 2 [20 ng/mL bFGF, 10 ng/mL hepatocyte growth factor (HGF), insulin selenium-transferrin (ITS; Life Technologies) and 0.61 g/L nicotinamide (Sigma)] and step 3 [20 ng/mL oncostatin M (OSM), 20 ng/mL HGF, 1% ITS, 0.61 g/L nicotinamide and 10−6 M dexamethasone (Dexa) (Sigma)] which lasted for 9 days each, 3 medium changes were performed each 3 days. The cells were microscopically followed at a regular basis. At the end of the maturation step, cells were harvested for analyses to appreciate the level of their phenotypic and functional differentiation. Control undifferentiated cells were kept during the whole process of differentiation in IMDM medium supplemented with 1% FCS.
For each HD of ADHLSC, the quality of differentiation was systematically evaluated at the morphological, genetic and functional level as previously documented (6,11). For the functional evaluation, CYP3A4 activity is mostly checked. The most recent related information is provided in the supplementary material from El-Kehdy et al. 2017.
Inflammatory priming
ADHLSCS were incubated overnight in medium supplemented with an inflammatory cytokine cocktail as previously described (20). This mix consists of 25 ng/mL IL-1β (Peprotech, Rocky Hill, NJ, USA) 103 U/mL IFN-γ, 50 ng/mL TNF-α, and 3×103 U/mL IFN-α (all from Prospec Inc., Rehovot, Israel) mostly expressed in inflammation sites (22).
Cytokinome analysis
Total mRNA was isolated from three sources: Hepatocytes, ADHLSC non-differentiated and differentiated using the TriPure Isolation Reagent (Roche Applied Science, Vilvoorde, Belgium). cDNA was obtained by reverse transcription of 1µg mRNA using qScript™ cDNA SuperMix (QUANTA bioscience, Gaithersburg, MD, USA) for 5 min at 25 °C, 30 min at 42 °C and 5 min at 85 °C. qPCR was performed on an ABI Prism® 7900HT Sequence Detection System (Applied Biosystem). We used 25 ng of cDNA in a qPCR with SYBR® Green PCR Master Mix (Applied Biosystems, Rotterdam, The Netherlands) and 0.32 µM of gene-specific forward and reverse primers. Primers specific for human cytokine mRNA were designed by using Primer Express 2.0 (Applied Biosystems) and can be found in Table S1. The following cytokines were tested: IL-1β, IL-6, IL-8, TNFα, CCL5, IL-12a, IL-12b, IL-23p19, IL-27p28 and Epstein-Barr virus-induced gene (EBI)-3, IL-1RA, TGF-β, IDO-1 and TDO-1.
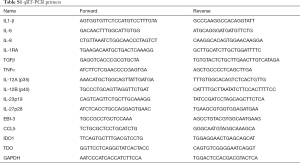
Full table
Statistical analysis
The influence of I and HD on ADHLSCs was presented as fold change. The comparison of HD ADHLSCs and hepatocytes was expressed as relative gene expression. Mann-Whitney test was used to analyze the statistical significance of the experimental results. P<0.05 was considered statistically significant, and all analyzes were performed with GraphPad Prism version 5.00 for windows (GraphPad Software, www.graphpad.com). The P values were obtained from primary data (before normalization) because P cannot be calculated for the data with the same rank.
Results
ADHLSC analysis
In this work, we first analyzed the expression of several cytokines in ADHLSCs and compared their expression levels with ADHLSCs that were differentiated into hepatocytes (HD ADHLSCs) and/or exposed to an inflammatory environment (ADHLSCs I). The gene expression values were normalized to those of GAPDH. Data are presented as the fold change in mRNA levels relative to the control (non-differentiated ADHLSCs) (Figure 1). The tested cytokines were classified into three families:
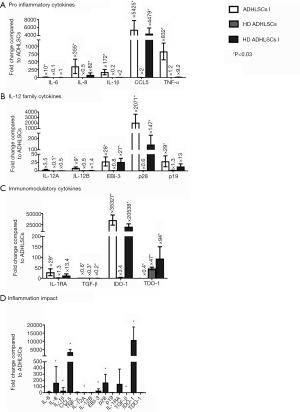
Pro-inflammatory cytokines (Figure 1A)
We evaluated the gene expression of the pro-inflammatory cytokines IL-1β, IL-6, IL-8, TNFα and CCL5. Concerning inflammation, the fold change was slightly increased for IL-6 (×10), moderately increased for IL-1β (×172) and IL-8 (×355), and highly increased for TNFα and CCL5 (×832 and ×5,425, respectively). All the effects of inflammatory cytokines in vitro were significant. Concerning the impact of HD, the majority of changes were not significant, but we noticed several trends. HD had a weak inverse effect on the expression of IL-6, IL-1β and IL-8 (×0.1, ×0.2 and ×0.5, respectively). On the other hand, HD slightly increased the expression of TNFα and CCL5. When the mixture of inflammatory cytokines was added, HD ADHLSCs showed increased expression of TNFα and IL-8 (×9.2 and ×82) and especially of CCL5 (×4,479). No effect on IL-1β or IL-6 (×2 and ×1, respectively) was observed relative to constitutive expression in ADHLSCs.
IL12 family cytokines (Figure 1B)
The gene expression of IL-12A (p35), IL-12B (p40), IL-23 (p19), IL-27 (p28) and EBI-3 was examined in ADHLSCs under four different conditions. After incubation with an inflammatory cocktail, the fold change was slightly increased for IL-12A (×3.5) and IL-12B (×9), moderately increased for EBI-3 (×28) and p19 (×29), and highly increased for p28 (×2,071). HD ADHLSCs showed a weak increase in p19 expression (×1.3). All these results were significant, except for IL-12A. Although most HD effects were not significant, we noticed several trends. HD slightly decreased the expression of IL-12A, IL-12B, p28 and EBI-3 (×0.1, ×0.5, ×0.6, and ×0.8, respectively). When the mixture of inflammatory cytokines was added, HD ADHLSCs showed increased expression of p19, EBI-3, and p28 (×13, ×27 and ×147, respectively). A small adverse effect was observed for IL-12A (×0.5). No effect on IL-12B (×1.4) was observed relative to constitutive expression in ADHLSCs.
Immunoregulatory cytokines (Figure 1C)
We also analyzed the gene expression of IL-1RA, TGFβ, IDO-1 and TDO-1 in ADHLSCs grown under four different conditions. Regarding inflammation, we observed a marked increase in IDO1 (×35,327) and a moderate increase in IL-1RA (×29) after adding the mixture of inflammatory cytokines. However, the expression of TGFβ and TDO1 (×0.6 and ×0.4, respectively) was decreased after the addition of the inflammation mixture. All these results were significant (P<0.05). Concerning HD, the majority of the changes were not significant, but we noticed several trends. HD caused a slight increase in IL-1RA and IDO1 expression (×1.3 and ×3.4, respectively) and a moderate effect on TDO1 expression (×47). On the other hand, HD slightly decreased the expression of TGFβ (×0.3). After the addition of inflammatory cytokines, HD ADHLSCs showed increased expression of IL-1RA and TDO1 (×13.4 and ×94, respectively) and very high expression of IDO1 (×20,538). A small adverse effect on TGFβ (×0.2) expression was observed relative to constitutive expression in ADHLSCs.
HD ADHLSCs compared to hepatocytes
Since inflammation impacts cytokine expression, we first studied cytokine modulation in hepatocytes and following the addition of inflammatory cytokines in vitro before analyzing the differences between cell types. These results are expressed as “relative gene expression” normalized to GAPDH. Hepatocytes do not constitutively express a majority of cytokines, except for IL-8 and CCL5, with moderate expression of EBI3 and TGFβ. We observed an increase in pro-inflammatory cytokines, such as IL-6, IL-8 and especially CCL5 (relative gene expression: 20,000), after adding the mixture of inflammatory cytokines. All these results were significant (P<0.05). However, we did not observe an increase in the expression of IL-1β or TNFα (Figure 2A). All cytokines in the IL-12 family were expressed only following the addition of the inflammation mixture, except for EBI3, which was present at low levels under classical conditions (P<0.05) (Figure 2B). Regarding the immunoregulatory cytokines, IDO1 and TDO1 were not detected under any condition. However, we observed a small, insignificant increase in TGFβ expression and an impressive increase in IL-1RA expression after the addition of inflammatory cytokines (Figure 2C).
We then evaluated the gene expression of cytokines in HD ADHLSCs compared to hepatocytes (Figure 3). Heterogenic modulations were observed: IL-1β and IL-6 were mostly expressed in HD ADHLSCs, whereas IL-8, CCL5 and TNFα were mostly expressed in hepatocytes (Figure 3A). Concerning the IL-12 family, no cytokine was constitutively expressed; however, three cytokines were mostly found in hepatocytes: IL-12A, p19 and EBI3. Other cytokines were not influenced by cell type. All of these results were significant (P<0.05) (Figure 3B). As previously observed, IDO1 was not expressed in these cells; however, we noticed slight expression of IL-1RA (P<0.05) (Figure 3C). We thus conclude that differences in cytokine expression exist between the two cell types.
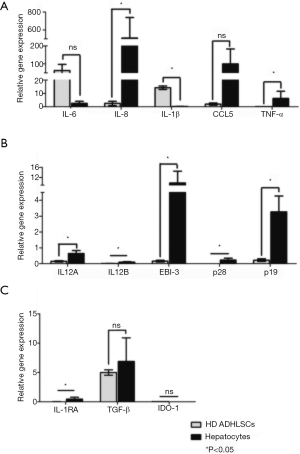
We next examined the cytokine profile of HD ADHLSCs under inflammatory conditions (Figure 4). Cytokines were most significantly expressed under inflammatory conditions, and we noted increased expression of IL-6, IL-8 and CCL5 in hepatocytes I (P=0.0286), while TNFα did not change significantly between cell types. IL-1β was weakly expressed in both cells, but we noted a significant decrease in its expression in hepatocytes I compared to HD ADHLSCs I (Figure 4A). We also observed that IL-12 cytokines were relatively weakly represented in HD ADHLSCs (<20), and increases in EBI3, p28 and p19 were observed in hepatocytes (Figure 4B). Finally, we observed weak expression of IDO1 in HD ADHLSCs following the addition of inflammatory cytokines but not in hepatocytes. We also observed a significant increase in IL-1RA, but no difference in TGFβ was noted between cell types (Figure 4C).
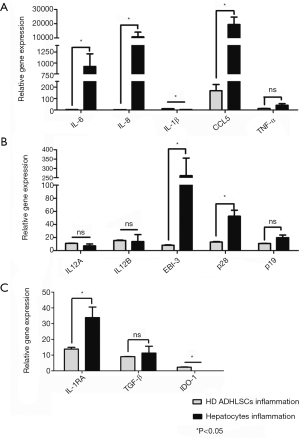
Discussion
The liver is essential for human survival and is currently considered the major metabolic organ due to its crucial roles in maintaining homeostasis. This organ is the site of several diseases (fibrosis, hepatitis and cirrhosis) that affect its function, leading to liver failure. The only current method used to treat these diseases is liver transplantation (30). However, the difficulty in finding donors, expensive cost of the transplant, numerous post-surgical complications and heavy immunosuppressive medication following the intervention make it necessary to find alternatives. Cell-based therapy is one possible method that can be used to regenerate liver cells (31,32). Current main objectives are to find hepatocyte-like progenitors with liver metabolic functions and cells able to modulate the cellular and molecular events associated with injury (33).
ADHLSCs have been reported as a new reservoir of hepatocytes (6). While expressing several mesenchymal markers as other mesenchymal stromal cells, these progenitor cells do not differentiate into osteoblasts and adipocytes but seem to undergo hepatocyte differentiation potential more efficiently than other MSC sources. As they display hepatocyte-like activities and are endowed with immunological features, they are considered a good candidate for liver tissue repair. To obtain an efficient therapeutic effect, there is a need to increase their engraftment and assure their long-term safety (24). Infusing ADHLSCs after extensive hepatectomy has improved cell engraftment and murine hepatic tissue regeneration, thereby confirming that ADHLSCs could be valuable for liver cell therapy (34). We previously showed that liver-based regenerative medicine using ADHLSCs is feasible and can lead to significant engraftment and parenchymal repopulation (18). Despite such favorable outcomes, the complex mechanisms underlying the long-term evolution of the graft structure remain to be fully understood, as shown by the high prevalence of progressive liver allograft fibrosis (35). Inflammation is a characteristic feature of several liver diseases and may differentially influence tissue repair and regeneration (29). Transplanted cells are sensitive to the environment and have to conjugate with resident or infiltrating immune cells to ensure safe therapeutic application (25,36,37). Indeed, cell engraftment was shown to critically depend on the inflammatory environment, which implies that progenitor cells such as ADHLSCs have to finely balance their immunological profile according to the context (28). It is thus crucial to evaluate the cytokines and growth factors implicated in liver function (38). We previously reported that inflammation may modulate the phenotype and expression pattern of toll-like receptors (TLRs) (17,39). Cytokines and growth factors are highly potent regulatory molecules that participate in the regulation of several immune functions and consequently play an important role in both immune recognition and allograft rejection (40-42). Here, we established the cytokinome of ADHLSCs in comparison to mature hepatocytes and ascertained the impact of an inflammatory cytokine mixture (I) and HD. We thus evaluated the gene expression profiles of three cytokine groups: pro-inflammatory cytokines (IL-1β, IL-6, IL-8, TNFα, CCL5), IL-12 family cytokines [IL-12a (p35), IL-12b (p40), IL-23 (p19), IL-27 (p28) and EBI-3], and immunoregulatory cytokines (IL-1RA, TGFβ, IDO-1 and TDO1). Immunomodulation may occur by the secretion of various types of cytokines that influence target immune cells either directly or indirectly by affecting the activities of regulatory immune cells (43). It should be pointed out that immunomodulatory cells are themselves subjects of cytokine regulation. During chronic or acute liver injury, the liver is a site of persistent inflammation and the immune response (44). Therefore, the success of liver cell therapy is linked to the capacity of immunomodulatory cells to manage both inflammation and hepatocyte apoptosis (45). Cytokines derived from MSCs have been highlighted in this field because they have a positive effect on hepatocyte proliferation and hepatic regeneration (15). ADHLSCs do not constitutively express pro-inflammatory cytokines or those belonging to the IL-12 family, while hepatic-differentiated ADHLSCs, especially those with inflammatory priming, exhibit significant expression of these cytokines. Concerning immunoregulatory cytokines, their expression was slightly increased within hepatocytes in an inflammatory context.
MSCs constitutively express IL-6, IL-1β, TGFβ, EBI3 and IL-12 (p35) mRNA. Following TLR stimulation, IL-23, IL-2, IL-1β, IL-6, and TGFβ were increased, whereas IL-12a was increased after inflammatory stimulation (39). Inflammation is believed to modulate the phenotype and expression patterns of TLRs in ADHLSCs) (17,39). Similar to MSCs, ADHLSCs likely sense and control inflammation, thus highlighting the dynamic interplay between innate and adaptive immune cell effectors (46).
IL-6 and TNFα are involved in the complex interplay between hepatic immune cells and hepatocytes (47). In our study, IL-6 was not influenced by HD and was expressed to a greater extent in hepatocytes. ADHLSCs demonstrated increased expression of IL-6 after inflammatory priming. IL-6 has been observed during hepatic diseases and its level is linked to disease progression (48). However, there are clear implications concerning its capacity to induce an acute phase response (47). IL-6 has been of special interest due to its capacity to protect hepatocytes (49).
TNFα belongs to a large family acting through the TNF receptor super family called “death receptors” (50). In this study, we proved that TNFα is modulated in vitro by the addition of an inflammatory cytokine mixture in ADHLSCs but not in hepatocytes. We also demonstrated no effect of HD in ADHLSCs. An elevated level of TNFα is associated with hepatocyte apoptosis (51) and characterizes patients with liver disease (48). TNFα can be secreted by MSCs, leading to a decrease in collagen 1 synthesis by HSCs (45). TNFα can also activate specific intracellular pathways in hepatocytes that influence cell fate in different manners (47).
IL-1β is secreted by different cell types, can cause the recruitment of inflammatory cells and is therefore involved in the immune response (52). The HD of ADHLSCs did not influence its expression. However, we observed a small effect of the inflammatory cytokine mixture in hepatocytes; IL-1β was more highly expressed in ADHLSCs than in hepatocytes. A previous review discussed the immunological importance and pivotal roles of IL-1 family cytokines in the development of acute and chronic liver diseases (53). Previous data have implicated IL-1β in improving the survival of MSCs after acute liver failure (54). Moreover, IL-1, by activating HSCs and inducing the production of metalloproteinases, may contribute to the progression of liver injury (55).
IL-8, another mediator of the immune reaction, can be produced by MSCs (56) and influences disease progression. In this study, IL-8 was influenced by the addition of a mixture of inflammatory cytokines in ADHLSCs and hepatocytes but not by HD. We also observed that this cytokine was more highly expressed in hepatocytes than in ADHLSCs. The interaction between MSCs and T lymphocytes notably induced an increase in IL-8 and CCL5, as previously demonstrated (57). IL-8 has been clearly linked to the evolution of chronic liver disease, where high levels of the cytokine have been found in the serum of patients with end-stage cirrhosis (58).
CCL5, which was first considered a T-cell product, is often found in inflammatory sites (59) and interacts with numerous receptors, including CCR1, 3 and 5. CCL5 expression was strongly increased by the inflammatory cytokine mixture in ADHLSCs and hepatocytes but not by the HD of ADHLSCs. This cytokine was more highly expressed in hepatocytes than in HD ADHLSCs. Co-culture of MSCs with T-cells induced increased CCL5 production (57). CCL5 and its receptor (CCR5) are substantially increased in patients with chronic liver diseases and may consequently represent therapeutic targets (60,61).
TGFβ, IL-12 (p40-35) and EBI3 are differentially expressed by different types of MSCs, and inflammatory priming may further increase their expression (62-64). IL-12 belongs to the complex “IL-12 family,” which also includes IL-23, IL-27 and IL-35 (65-67). These different members share several subunits: IL-12 (p35/p40), IL-23 (p19/p40), IL-27 (p28/EBI3) and IL-35 (p35/EBI3) (68,69). These cytokines are involved in T helper differentiation and functions (70). Since they are found in the serum of patients with chronic liver diseases, IL-12 cytokines could be used as an immunological biomarker (71). In this work, we observed that this family is not constitutively expressed by ADHLSCs and is not influenced by HD. However, we observed a clear increase in these cytokines following the addition of the inflammatory cytokine mixture (especially EBI-3, p19 and p28), and all were expressed to a greater extent in hepatocytes than in HD ADHLSCs. IL-27, a soluble factor that regulates antigen-presenting cells and T-cell function, is highly expressed by MSCs (66,72). In liver injury, this cytokine has been associated with pro-inflammatory effects and is thus positively correlated with Th17 cell function (73). Finally, IL-27 from human MSCs subjected to HD plays an important role in liver recovery in mouse models (74). In contrast to IL-27, which is not hepatoprotective, the therapeutic cytokine IL-30 (IL-27 p28) has been proposed to suppress pro-inflammatory cytokine-associated liver toxicity (66). IL-23 (p19) and IL-27 (p28) were not affected by inflammation in WJ-MSCs in contrast to AT- and BM-MSCs (62).
IL-1 receptor antagonist (IL-1RA) is an important anti-inflammatory agent (75) and is constitutively expressed by AT-MSCs (62). The biological functions of IL-1 are negatively regulated by IL-1RA, i.e., it competes with IL-1α, and IL-1β may also bind to the IL-1 receptor (IL-1R1). IL-1RA expression was increased by the addition of the inflammatory mixture and was significantly higher in hepatocytes than in HD ADHLSCs. IL1-RA may correct liver dysfunction and promote liver regeneration by preventing hepatocyte apoptosis and increasing cell proliferation (76,77).
TGFβ is widely expressed and has been implicated in liver disease progression (78). This cytokine is an inhibitor of hepatocyte proliferation and may affect the pathogenesis of fibrosis in liver diseases (78,79). In ADHLSCs, this cytokine was not affected by the inflammation mixture or HD, and it was more highly expressed in ADHLSCs than in hepatocytes. Aberrant signaling of TGF-β and IL-6 may play a role in the cellular differentiation of hepatic progenitor/stem cells and hepatocellular carcinoma (66).
Indoleamine-2,3-dioxygenase (IDO) and tryptophan-2,3-dioxygenase (TDO) have been thoroughly investigated in the context of inflammatory diseases (80). IDO1 can be induced in MSCs following a pro-inflammatory signal and subsequently suppresses the T-cell response by depleting tryptophan (81). IDO1 was clearly increased by the inflammatory cytokine mixture in ADHLSCs but not by HD. Moreover, it is not expressed in hepatocytes, whereas TDO1 is. TDO1 was increased following HD but not after the addition of the inflammatory cytokine mixture. IDO1 deficiency has been proven to promote liver regeneration after hepatectomy (82). TDO1, which controls energy homeostasis, is constitutively expressed by liver cells (83).
The liver is thus constantly subjected to different attacks, causing immune cell activation and inflammation, as well as continuous rounds of necrosis and hepatocyte regeneration (66). Such conditions make a permissive environment for the development of fibrosis and cirrhosis and the expansion/alteration of the stem cell compartment. Cytokines and cytokine-induced inflammatory responses are the primary factors for controlling whether the liver undergoes effective repair and regeneration or degeneration, oncosis, and fibrosis. Evidence suggests that cytokines, by directing the behavior of resident endogenous cells, play a major role in the repair response generated during cell-based therapies (84). A multitude of cytokines secreted from MSCs confer their multifunctional therapeutics, but their overall spectrum and respective expression strength have not been thoroughly illustrated (85). The contribution of cytokines and chemokines to the hepatocytic response in liver homeostasis and injury could be beneficial for the treatment of liver diseases (50). In parallel, treatment of MSCs derived from human umbilical cords (ucMSCs) has shown improvements in immunoregulatory capacity and immunogenicity, which may improve the efficacy of ucMSCs as an immunotherapy targeted towards liver inflammation (86). ADHLSCs responded to the inflammatory cytokine mixture by adjusting their cytokine patterns. The development of liver cell therapy based on the use of ADHLSCs should be well-monitored regarding immunological and inflammatory features.
Conclusions
Liver regeneration is thus a process tightly coordinated by hepatocytes and non-parenchymal cells. However, inflammation may alter the well-ordered phenotypic changes of non-parenchymal cells by creating an inflammatory environment, including an immune-active niche. Therefore, these effects may lead to an aberrant healing process, i.e., liver fibrosis. Further investigation of the mechanisms underlying liver immunobiology and the role of stem/progenitor cells in regeneration will aid in the development of therapeutic strategies to counter liver disease.
Acknowledgements
None.
Footnote
Conflicts of Interest: The authors have no conflicts of interest to declare.
Ethical Statement: The study was approved by ethics committee of the Jules Bordet Institute and Université Libre de Bruxelles (ULB) and ethics committees of the St-Luc Hospital and faculty of Medicine of the Université Catholique de Louvain (UCL).
References
- Itri JN, Heller MT, Tublin ME. Hepatic transplantation: postoperative complications. Abdom Imaging 2013;38:1300-33. [Crossref] [PubMed]
- Iansante V, Mitry RR, Filippi C, et al. Human hepatocyte transplantation for liver disease: current status and future perspectives. Pediatr Res 2018;83:232-40. [Crossref] [PubMed]
- Gramignoli R, Vosough M, Kannisto K, et al. Clinical hepatocyte transplantation: practical limits and possible solutions. Eur Surg Res 2015;54:162-77. [Crossref] [PubMed]
- Yu Y, Fisher JE, Lillegard JB, et al. Cell therapies for liver diseases. Liver Transpl 2012;18:9-21. [Crossref] [PubMed]
- Forbes SJ, Gupta S, Dhawan A. Cell therapy for liver disease: From liver transplantation to cell factory. J Hepatol 2015;62:S157-S169. [Crossref] [PubMed]
- Najimi M, Khuu DN, Lysy PA, et al. Adult-derived human liver mesenchymal-like cells as a potential progenitor reservoir of hepatocytes? Cell Transplant 2007;16:717-28. [Crossref] [PubMed]
- Campagnoli C, Roberts IA, Kumar S, et al. Identification of mesenchymal stem/progenitor cells in human first-trimester fetal blood, liver, and bone marrow. Blood 2001;98:2396-402. [Crossref] [PubMed]
- in 't Anker PS, Noort WA, Scherjon SA, et al. Mesenchymal stem cells in human second-trimester bone marrow, liver, lung, and spleen exhibit a similar immunophenotype but a heterogeneous multilineage differentiation potential. Haematologica 2003;88:845-52. [PubMed]
- Larijani B, Aghayan HR, Goodarzi P, et al. GMP-grade human fetal liver-derived mesenchymal stem cells for clinical transplantation. Methods Mol Biol 2015;1283:123-36. [Crossref] [PubMed]
- Pan Q, Fouraschen SMG, Kaya FSFA, et al. Mobilization of hepatic mesenchymal stem cells from human liver grafts. Liver Transpl 2011;17:596-609. [Crossref] [PubMed]
- Khuu DN, Scheers I, Ehnert S, et al. In vitro differentiated adult human liver progenitor cells display mature hepatic metabolic functions: a potential tool for in vitro pharmacotoxicological testing. Cell Transplant 2011;20:287-302. [Crossref] [PubMed]
- Berardis S, Dwisthi Sattwika P, Najimi M, et al. Use of mesenchymal stem cells to treat liver fibrosis: current situation and future prospects. World J Gastroenterol 2015;21:742-58. [Crossref] [PubMed]
- Berardis S, Lombard C, Evraerts J, et al. Gene expression profiling and secretome analysis differentiate adult-derived human liver stem/progenitor cells and human hepatic stellate cells. PLoS One 2014;9. [Crossref] [PubMed]
- Khuu DN, Nyabi O, Maerckx C, et al. Adult human liver mesenchymal stem/progenitor cells participate in mouse liver regeneration after hepatectomy. Cell Transplant 2013;22:1369-80. [Crossref] [PubMed]
- Fouraschen SMG, Pan Q, de Ruiter PE, et al. Secreted factors of human liver-derived mesenchymal stem cells promote liver regeneration early after partial hepatectomy. Stem Cells Dev 2012;21:2410-9. [Crossref] [PubMed]
- Dollet PE, Ravau J, Andre F, et al. Comprehensive Screening of Cell Surface Markers Expressed by Adult-Derived Human Liver Stem/Progenitor Cells Harvested at Passage 5: Potential Implications for Engraftment. Stem Cells Int 2016;2016. [Crossref] [PubMed]
- Raicevic G, Najar M, Najimi M, et al. Influence of inflammation on the immunological profile of adult-derived human liver mesenchymal stromal cells and stellate cells. Cytotherapy 2015;17:174-85. [Crossref] [PubMed]
- Sokal EM, Stephenne X, Ottolenghi C, et al. Liver engraftment and repopulation by in vitro expanded adult derived human liver stem cells in a child with ornithine carbamoyltransferase deficiency. JIMD Rep 2014;13:65-72. [Crossref] [PubMed]
- Sokal EM. Treating inborn errors of liver metabolism with stem cells: current clinical development. J Inherit Metab Dis 2014;37:535-9. [Crossref] [PubMed]
- Defresne F, Tondreau T, Stephenne X, et al. Biodistribution of adult derived human liver stem cells following intraportal infusion in a 17-year-old patient with glycogenosis type 1A. Nucl Med Biol 2014;41:371-5. [Crossref] [PubMed]
- Sokal EM, Lombard CA, Roelants V, et al. Biodistribution of Liver-Derived Mesenchymal Stem Cells After Peripheral Injection in a Hemophilia A Patient. Transplantation 2017;101:1845-51. [Crossref] [PubMed]
- Najimi M, Berardis S, El-Kehdy H, et al. Human liver mesenchymal stem/progenitor cells inhibit hepatic stellate cell activation: in vitro and in vivo evaluation. Stem Cell Res Ther 2017;8:131. [Crossref] [PubMed]
- Nicolas C, Wang Y, Luebke-Wheeler J, et al. Stem Cell Therapies for Treatment of Liver Disease. Biomedicines 2016;4. [Crossref] [PubMed]
- Sharpe ME, Morton D, Rossi A. Nonclinical safety strategies for stem cell therapies. Toxicol Appl Pharmacol 2012;262:223-31. [Crossref] [PubMed]
- Pluchino S, Cossetti C. How stem cells speak with host immune cells in inflammatory brain diseases. Glia 2013;61:1379-401. [Crossref] [PubMed]
- Le Blanc K, Davies LC. Mesenchymal stromal cells and the innate immune response. Immunol Lett 2015;168:140-6. [Crossref] [PubMed]
- Seki E, Schwabe RF. Hepatic inflammation and fibrosis: functional links and key pathways. Hepatology 2015;61:1066-79. [Crossref] [PubMed]
- El-Kehdy H, Sargiacomo C, Fayyad-Kazan M, et al. Immunoprofiling of Adult-Derived Human Liver Stem/Progenitor Cells: Impact of Hepatogenic Differentiation and Inflammation. Stem Cells Int 2017;2017. [Crossref] [PubMed]
- Najar M, Fayyad-Kazan H, Faour WH, et al. Human hepatic stellate cells and inflammation: A regulated cytokine network balance. Cytokine 2017;90:130-4. [Crossref] [PubMed]
- Coppin L, Sokal E, Stephenne X. Hepatocyte Transplantation in Children. Methods Mol Biol 2017;1506:295-315. [Crossref] [PubMed]
- Saidi RF, Kenari SKH. Liver ischemia/reperfusion injury: an overview. J Invest Surg 2014;27:366-79. [Crossref] [PubMed]
- Jang YO, Jun BG, Baik SK, et al. Inhibition of hepatic stellate cells by bone marrow-derived mesenchymal stem cells in hepatic fibrosis. Clin Mol Hepatol 2015;21:141-9. [Crossref] [PubMed]
- Sokal EM. From hepatocytes to stem and progenitor cells for liver regenerative medicine: advances and clinical perspectives. Cell Prolif 2011;44:39-43. [Crossref] [PubMed]
- Herrero A, Prigent J, Lombard C, et al. Adult-Derived Human Liver Stem/Progenitor Cells Infused 3 Days Postsurgery Improve Liver Regeneration in a Mouse Model of Extended Hepatectomy. Cell Transplant 2017;26:351-64. [Crossref] [PubMed]
- Venturi C, Sempoux C, Quinones JA, et al. Dynamics of allograft fibrosis in pediatric liver transplantation. Am J Transplant 2014;14:1648-56. [Crossref] [PubMed]
- Charron D. Allogenicity & immunogenicity in regenerative stem cell therapy. Indian J Med Res 2013;138:749-54. [PubMed]
- Sana G, Lombard C, Vosters O, et al. Adult human hepatocytes promote CD4(+) T-cell hyporesponsiveness via interleukin-10-producing allogeneic dendritic cells. Cell Transplant 2014;23:1127-42. [Crossref] [PubMed]
- Tilg H. Cytokines and liver diseases. Can J Gastroenterol 2001;15:661-8. [Crossref] [PubMed]
- Raicevic G, Rouas R, Najar M, et al. Inflammation modifies the pattern and the function of Toll-like receptors expressed by human mesenchymal stromal cells. Hum Immunol 2010;71:235-44. [Crossref] [PubMed]
- Feghali CA, Wright TM. Cytokines in acute and chronic inflammation. Front Biosci 1997;2:d12-d26. [Crossref] [PubMed]
- Benjamin LC, Allan JS, Madsen JC. Cytokines in immunity and allograft rejection. Crit Rev Immunol 2002;22:269-79. [PubMed]
- Lowes KN, Croager EJ, Olynyk JK, et al. Oval cell-mediated liver regeneration: Role of cytokines and growth factors. J Gastroenterol Hepatol 2003;18:4-12. [Crossref] [PubMed]
- Kyurkchiev D, Bochev I, Ivanova-Todorova E, et al. Secretion of immunoregulatory cytokines by mesenchymal stem cells. World J Stem Cells 2014;6:552-70. [Crossref] [PubMed]
- Meier RPH, Muller YD, Morel P, et al. Transplantation of mesenchymal stem cells for the treatment of liver diseases, is there enough evidence? Stem Cell Res 2013;11:1348-64. [Crossref] [PubMed]
- Parekkadan B, van Poll D, Megeed Z, et al. Immunomodulation of activated hepatic stellate cells by mesenchymal stem cells. Biochem Biophys Res Commun 2007;363:247-52. [Crossref] [PubMed]
- Bernardo ME, Fibbe WE. Mesenchymal stromal cells: sensors and switchers of inflammation. Cell Stem Cell 2013;13:392-402. [Crossref] [PubMed]
- Tacke F, Luedde T, Trautwein C. Inflammatory pathways in liver homeostasis and liver injury. Clin Rev Allergy Immunol 2009;36:4-12. [Crossref] [PubMed]
- Streetz KL, Tacke F, Leifeld L, et al. Interleukin 6/gp130-dependent pathways are protective during chronic liver diseases. Hepatology 2003;38:218-29. [Crossref] [PubMed]
- Kovalovich K, Li W, DeAngelis R, et al. Interleukin-6 protects against Fas-mediated death by establishing a critical level of anti-apoptotic hepatic proteins FLIP, Bcl-2, and Bcl-xL. J Biol Chem 2001;276:26605-13. [Crossref] [PubMed]
- Walczak H. Death receptor-ligand systems in cancer, cell death, and inflammation. Cold Spring Harb Perspect Biol 2013;5. [Crossref] [PubMed]
- Rimkunas VM, Graham MJ, Crooke RM, et al. TNF-{alpha} plays a role in hepatocyte apoptosis in Niemann-Pick type C liver disease. J Lipid Res 2009;50:327-33. [Crossref] [PubMed]
- Kay J, Calabrese L. The role of interleukin-1 in the pathogenesis of rheumatoid arthritis. Rheumatology (Oxford) 2004;43:iii2-iii9. [Crossref] [PubMed]
- Tsutsui H, Cai X, Hayashi S. Interleukin-1 Family Cytokines in Liver Diseases. Mediators Inflamm 2015;2015. [Crossref] [PubMed]
- Ma H, Shi X, Yuan X, et al. IL-1beta siRNA adenovirus benefits liver regeneration by improving mesenchymal stem cells survival after acute liver failure. Ann Hepatol 2016;15:260-70. [PubMed]
- Gieling RG, Wallace K, Han YP. Interleukin-1 participates in the progression from liver injury to fibrosis. Am J Physiol Gastrointest Liver Physiol 2009;296:G1324-G1331. [Crossref] [PubMed]
- Wang J, Wang Y, Wang S, et al. Bone marrow-derived mesenchymal stem cell-secreted IL-8 promotes the angiogenesis and growth of colorectal cancer. Oncotarget 2015;6:42825-37. [Crossref] [PubMed]
- Najar M, Raicevic G, Fayyad-Kazan H, et al. Impact of different mesenchymal stromal cell types on T-cell activation, proliferation and migration. Int Immunopharmacol 2013;15:693-702. [Crossref] [PubMed]
- Zimmermann HW, Seidler S, Gassler N, et al. Interleukin-8 is activated in patients with chronic liver diseases and associated with hepatic macrophage accumulation in human liver fibrosis. PLoS One 2011;6. [Crossref] [PubMed]
- Appay V, Dunbar PR, Cerundolo V, et al. RANTES activates antigen-specific cytotoxic T lymphocytes in a mitogen-like manner through cell surface aggregation. Int Immunol 2000;12:1173-82. [Crossref] [PubMed]
- Mohs A, Kuttkat N, Reissing J, et al. Functional role of CCL5/RANTES for HCC progression during chronic liver disease. J Hepatol 2017;66:743-53. [Crossref] [PubMed]
- Berres ML, Koenen RR, Rueland A, et al. Antagonism of the chemokine Ccl5 ameliorates experimental liver fibrosis in mice. J Clin Invest 2010;120:4129-40. [Crossref] [PubMed]
- Raicevic G, Najar M, Stamatopoulos B, et al. The source of human mesenchymal stromal cells influences their TLR profile as well as their functional properties. Cell Immunol 2011;270:207-16. [Crossref] [PubMed]
- Crop MJ, Baan CC, Korevaar SS, et al. Inflammatory conditions affect gene expression and function of human adipose tissue-derived mesenchymal stem cells. Clin Exp Immunol 2010;162:474-86. [Crossref] [PubMed]
- Hemeda H, Jakob M, Ludwig AK, et al. Interferon-gamma and tumor necrosis factor-alpha differentially affect cytokine expression and migration properties of mesenchymal stem cells. Stem Cells Dev 2010;19:693-706. [Crossref] [PubMed]
- Aggarwal S, Ghilardi N, Xie MH, et al. Interleukin-23 promotes a distinct CD4 T cell activation state characterized by the production of interleukin-17. J Biol Chem 2003;278:1910-4. [Crossref] [PubMed]
- Dibra D, Cutrera J, Xia X, et al. Interleukin-30: a novel antiinflammatory cytokine candidate for prevention and treatment of inflammatory cytokine-induced liver injury. Hepatology 2012;55:1204-14. [Crossref] [PubMed]
- Trinchieri G. Interleukin-12 and interferon-gamma. Do they always go together? Am J Pathol 1995;147:1534-8. [PubMed]
- Oppmann B, Lesley R, Blom B, et al. Novel p19 protein engages IL-12p40 to form a cytokine, IL-23, with biological activities similar as well as distinct from IL-12. Immunity 2000;13:715-25. [Crossref] [PubMed]
- Ringkowski S, Thomas PS, Herbert C. Interleukin-12 family cytokines and sarcoidosis. Front Pharmacol 2014;5:233. [Crossref] [PubMed]
- Heufler C, Koch F, Stanzl U, et al. Interleukin-12 is produced by dendritic cells and mediates T helper 1 development as well as interferon-gamma production by T helper 1 cells. Eur J Immunol 1996;26:659-68. [Crossref] [PubMed]
- El-Emshaty HM, Nasif WA, Mohamed IE. Serum Cytokine of IL-10 and IL-12 in Chronic Liver Disease: The Immune and Inflammatory Response. Dis Markers 2015;2015. [Crossref] [PubMed]
- Silva WAJ, Covas DT, Panepucci RA, et al. The profile of gene expression of human marrow mesenchymal stem cells. Stem Cells 2003;21:661-9. [Crossref] [PubMed]
- Zhang GL, Xie DY, Ye YN, et al. High level of IL-27 positively correlated with Th17 cells may indicate liver injury in patients infected with HBV. Liver Int 2014;34:266-73. [Crossref] [PubMed]
- Zagoura DS, Roubelakis MG, Bitsika V, et al. Therapeutic potential of a distinct population of human amniotic fluid mesenchymal stem cells and their secreted molecules in mice with acute hepatic failure. Gut 2012;61:894-906. [Crossref] [PubMed]
- Ortiz LA, Dutreil M, Fattman C, et al. Interleukin 1 receptor antagonist mediates the antiinflammatory and antifibrotic effect of mesenchymal stem cells during lung injury. Proc Natl Acad Sci U S A 2007;104:11002-7. [Crossref] [PubMed]
- Francois S, Mouiseddine M, Allenet-Lepage B, et al. Human mesenchymal stem cells provide protection against radiation-induced liver injury by antioxidative process, vasculature protection, hepatocyte differentiation, and trophic effects. Biomed Res Int 2013;2013. [Crossref] [PubMed]
- Conrad E, Resch TK, Gogesch P, et al. Protection against RNA-induced liver damage by myeloid cells requires type I interferon and IL-1 receptor antagonist in mice. Hepatology 2014;59:1555-63. [Crossref] [PubMed]
- Milani S, Herbst H, Schuppan D, et al. Transforming growth factors beta 1 and beta 2 are differentially expressed in fibrotic liver disease. Am J Pathol 1991;139:1221-9. [PubMed]
- Fausto N, Mead JE, Gruppuso PA, et al. Effects of TGF-beta s in the liver: cell proliferation and fibrogenesis. Ciba Found Symp 1991;157:165-74. [PubMed]
- Ball HJ, Jusof FF, Bakmiwewa SM, et al. Tryptophan-catabolizing enzymes - party of three. Front Immunol 2014;5:485. [Crossref] [PubMed]
- Grohmann U, Fallarino F, Puccetti P. Tolerance, DCs and tryptophan: much ado about IDO. Trends Immunol 2003;24:242-8. [Crossref] [PubMed]
- Ogiso H, Ito H, Kanbe A, et al. The Inhibition of Indoleamine 2,3-Dioxygenase Accelerates Early Liver Regeneration in Mice After Partial Hepatectomy. Dig Dis Sci 2017;62:2386-96. [Crossref] [PubMed]
- Knox WE, Mehler AH. The conversion of tryptophan to kynurenine in liver. I. The coupled tryptophan peroxidase-oxidase system forming formylkynurenine. J Biol Chem 1950;187:419-30. [PubMed]
- Stoddart MJ. Mesenchymal Stem Cells as a Source of Repair Cytokines: Mesenchymal Stem Cells as the Conductor. J Am Acad Orthop Surg 2015;23:452-3. [Crossref] [PubMed]
- Park CW, Kim KS, Bae S, et al. Cytokine secretion profiling of human mesenchymal stem cells by antibody array. Int J Stem Cells 2009;2:59-68. [Crossref] [PubMed]
- de Witte SFH, Merino AM, Franquesa M, et al. Cytokine treatment optimises the immunotherapeutic effects of umbilical cord-derived MSC for treatment of inflammatory liver disease. Stem Cell Res Ther 2017;8:140. [Crossref] [PubMed]