Adipose tissue induces a better liver regeneration after living liver donation in normal weight donors
Highlight box
Key findings
• Subcutaneous adipose tissue is a positive predictive factor to estimate the goodness of liver regeneration after partial hepatectomy in normostenic donors.
What is known and what is new?
• Regenerating liver hepatocytes after partial hepatectomy in rats is known to normally accumulate fat microdroplets temporarily. This accumulation disappears spontaneously.
• In this study, we investigated the positive impact of body adipose tissue on liver regeneration. We found that living donors with a higher fat mass index had a better liver volume gain after surgery.
What is the implication, and what should change now?
• A nutritional evaluation and supplementation before liver surgery could positively influence the liver regeneration capacity after surgery and therewith the postoperative outcome consequently.
• Prospective studies with histological and liver functional aspects are required to better understand the relationship between regenerating liver and adipose tissue.
Introduction
The fact learned from clinical practice that the liver regenerates differently after taking into account the remaining size from one patient to another is not yet completely understood. A better understanding of the factors affecting liver regeneration is of great clinical importance since the quality of liver regeneration determines the outcome after major liver resection (1).
On the one hand, hepatic steatosis impairs liver regeneration after injury (2), and the accumulation of fat droplets in hepatocytes blocks liver regeneration (3). On the other hand, regenerating liver hepatocytes normally accumulate fat microdroplets according to Michalopoulos et al. (4) This transient fatty liver occurs in rats from 24 to 72 h after partial hepatectomy (PHx) and disappears spontaneously (4). There is also a rather pronounced accumulation of triglycerides in hepatocytes from 20 to 72 hours after PHx associated with a marked induction of lipogenic enzymes (5). Studies have shown that this phenomenon is an essential component of the regenerative process (5). Further evidence supporting the protective effect of fatty acid supplementation following partial hepatectomy was provided by Yan et al. (6). According to that study, the supplementation of rats with fish oil, which contains n-3 polyunsaturated fatty acids (n-3 PUFAs), may promote liver regeneration after 70% partial hepatectomy and protect the postoperative hepatic function, representing a protective agent against liver failure.
Summarizing the abovementioned findings, this process is thought to represent a metabolic adaptation of hepatocytes so that the emerging new cells have readily available energy as well as materials they can use to build cellular membranes. Therefore, the amount of adipose tissue and its distribution could be an important factor affecting liver regeneration after partial hepatectomy. This protective effect can be derived from the already existing body fat content, or it can be supported by alimentary supplementation perioperatively.
Accordingly, we supposed that the adipose tissue plays a positive role in generating liver and should induce a better liver regeneration after resection. The aim of this study was to evaluate the potential role of body fat tissue in liver regeneration capacity after major liver resection or split liver transplantation in a cohort of living donors and their recipients. We present this article in accordance with the STROBE reporting checklist (available at https://hbsn.amegroups.com/article/view/10.21037/hbsn-22-442/rc).
Methods
The study was conducted in accordance with the Declaration of Helsinki (as revised in 2013) (7). The study was initiated after approval by the local ethics committee of Friedrich-Schiller-University, Jena (registration number 3826-07/13) and written informed consent was obtained from the individual patients. In our study, we observed liver regeneration in 70 living donors who underwent right hepatectomy (Segment V to VIII) and 50 recipients who underwent living donation right graft liver transplantation (Segment V to VIII) at Jena University Hospital between 2013 and 2022. Twenty recipients had to be excluded, either since the computed tomography was not done at an adequate time after surgery, or because of severe postoperative course, which supposedly influences the liver regeneration remarkedly. The parenchymal transection was performed using CUSA “Cavitron Ultrasonic Surgical Aspirator” (Valleylab Boulder, CO, USA). There were no strict defined selection criteria for donors, but we include only healthy donors with ASA grade I (American Society of Anesthesiology) after an extensive medical evaluation. The matter of age and body mass index (BMI) was considered individually, respecting the health state of the donor. WHO classification was considered to determine the overweight/obesity state [normal weight: BMI (kg/m2) >18 to <25, overweight: ≥25 to <30, obesity class 1: ≥30 to <35, class 2: ≥35 to <40, class 3: ≥40]. During the evaluation procedure, thin-layer (0.625 mm) three-phase contrast-enhanced computed tomography and magnetic resonance tomography of the liver together with magnetic resonance cholangiography were performed. The liver volume assay was performed based on the computed tomography images with special computer software (Synapse 3D, FUJIFILM, Tokyo, Japan). This program offers the possibility to measure different liver volumes, allowing to estimate the future liver remnant volume (FLR). A selective volume measurement is possible in different ways, either with free-hand extraction of a specific liver area (e.g., the right liver lobe) or measurements based on a portal venous or venous vascular tree. Another utility offered by the same software is the body fat analysis. This can be assessed using contrast-enhanced computed tomography studies at the venous phase. The margins of the study area are standardized at tenth thoracic vertebra as the upper margin and the pubic symphysis as the lower margin. Depending on the Hounsfield unit threshold, which is assessed from the subcutaneous adipose tissue, the subcutaneous and visceral fat mass are calculated automatically. This software detects adipose tissue in the stem based on the Hounsfield unit assessed from the subcutaneous space. This could automatically lead to a better assessment of subcutaneous adipose tissue. Since these values alone have reduced validity, we considered a fatty mass variable related to body mass in our statistical analysis. This can be calculated, complying with the calculation of BMI, through the following formula:
Stratification can be performed to determine the subcutaneous (sFMI) and visceral (vFMI) fat indices. Fat analysis was performed within the donor evaluation phase preoperatively. Moreover, the software can calculate the mass of the psoas muscle so that this was considered too in our statistical analysis to show the potential relationship between psoas mass and liver regeneration as a secondary endpoint. To evaluate the liver regeneration capacity, the liver volume assay was performed at three time points: preoperatively within the donor evaluation phase, early postoperatively within the first two weeks after donation, and late postoperatively six months after donation. The late measurement was performed just in 42 donors and 42 recipients. The rest of them does not present at the late observation because of logistical challenges, especially the far distance to study center. The percental liver volume gain was considered since the absolute liver volume gain has low validity. The following formula was used to estimate the percent volume gain:
n = time of volume measurement:
- 0 for the baseline measurement prior to donation
- 1 for the early measurement
- 2 for the late measurement
LiMax was used to evaluate liver function and its relationship to the estimated volumes in our cohort. This is since 2009 in clinical use as a bedside real-time liver function test (8). During the LiMAx test, 13C-Metacetine is given intravenously. The 13C-Metacetine will be converted in the liver to 13CO2, which will be exhaled and measured in the expired air. This will be represented through a weight and time adapted value (µg/kg/h), which reflects the quality of enzymatic liver function. Prothrombin time and Bilirubin trends were analyzed for further evaluating of liver function after surgery.
Statistical analysis
The statistical analysis was performed with IBM SPSS Statistics 25 software (IBM, Armonk, New York, USA). First, box plots and Gaussian bell curves were used to evaluate the central tendency and normal distribution of FLR regeneration and FMI in our cohort. A t-test was used to compare the central tendency of the liver volumes between the three time points. Thereafter, Pearson’s correlation was used to examine the potential correlation between the adipose tissue and liver regeneration. In the case of Pearson’s correlation, a verification of linearity between the independent and dependent variables was performed with the aid of a scatter plot.
To determine how large the found correlation is, we referred to Cohen’s classification (1992) as follows:
Since the distribution of adipose tissue usually differs in males and females, the ratio of vFMI/sFMI was compared accordingly using Mann-Whitney-U-test. Thereafter, the correlation studies between adipose tissue and FLR-gain were made in stratification according to gender.
With the aim of better exposition, further correlating independent variables such as age and regeneration period were then analyzed in a linear regression model, considering the Gauss-Markov theorem. The significance level was determined with a P value <0.05.
Results
Descriptive statistics
The median age of the donors in our study cohort was 43.5 years (range, 22–60 years). 41 donors were women, and 29 were men. The median age of the recipients was 54 years (range, 21–71 years). Eight recipients were women, and 42 were men. The median BMI was in donors 24.3 kg/m2 (range, 18.8–34.1 kg/m2), and in recipients 25.6 kg/m2 (range, 17.3–36.3 kg/m2). In total 65 (54.2%) patients had normal weight, 41 (34.2%) overweight and 14 (11.7%) class 1 obesity. 43 (61.4) donors had normal weight, 21 (30%) overweight and 6 (8.6%) class 1 obesity. 22 (44%) recipients had normal weight, 20 (40%) overweight and 8 (16%) class 1 obesity. The median percentage volume gain was in donors 193% (range, 126–270%) in a median interval of 10 days (range, 4–21 days) and 228.1% (range, 184.3–354.2%) in a median interval of 28 weeks (range, 21–39 weeks). In recipients, the median percental volume gain was 169% (range, 121–259%) in a median interval of 10 days (range, 5–14 days) and 181.7% (range, 113.6–277.3%) in a median interval of 27 weeks (range, 18–40 weeks). Basic cohort characteristics and descriptive statistics of the investigated variables are provided per Table 1.
Table 1
Variable | Total, n=120 | Donors, n=70 | Recipients, n=50 |
---|---|---|---|
Age (years) | 50; 21–71 | 43.5; 22–60 | 54; 21–71 |
Sex | m =71, w =49 | m =29, w =41 | m =42, w =8 |
Height (m) | 1.74; 1.46–1.96 | 1.71; 1.55–1.96 | 1.76; 1.46–1.90 |
Weight (kg) | 76; 53–110 | 75; 53–107 | 77.5; 55–110 |
BMI (kg/m2) | 24.6; 17.3–36.3 | 24.3; 18.8–34.1 | 25.6; 17.3–36.3 |
TLV (mL) | 1,417; 1,090–2,040 | 1,431; 1,090–2,040 | 1,410; 1,090–2,040 |
FLR0 (mL) | 625.5; 324–1,297 | 511.5; 324–802 | 932; 630–1,297 |
FLR0 (%) | 40; 25.7–74.3 | 36; 25.7–43.9 | 63.7; 56.1–74.3 |
GBWR0 (%) | 0.84; 0.43–1.95 | 0.7; 0.43–1.1 | 1.82; 0.70–1.95 |
Interval1 (d) | 10; 4–21 | 10; 4–21 | 10; 5–14 |
FLR1 (mL) | 1,190.5; 544–1,968 | 970.5; 544–1,663 | 1,560.5; 1,194–1,968 |
GBWR1 (%) | 1.56; 0.88–3.19 | 1.37; 0.88–1.94 | 1.99; 1.36–3.19 |
Interval2 (w) | 27; 18–40 | 28; 21–39 | 27; 18–40 |
FLR2 (mL) | 1,401; 770–2,449 | 1,205; 770–2,054 | 1,679.5; 1,168–2,449 |
GBWR2 (%) | 1.91; 1.03–3.12 | 1.62; 1.03–2.38 | 2.17; 1.24–3.12 |
Gain1 (mL) | 502.5; 179–1,074 | 467; 179–861 | 613; 224–1,074 |
Gain2 (mL) | 708; 159–1,464 | 677.5; 395–1,252 | 741.5; 159–1,464 |
Gain1 (%) | 179.8; 121.2–270.2 | 193; 126–270.2 | 169; 121.2–258.9 |
Gain2 (%) | 209; 113.6–345.2 | 228.1; 184.3–354.2 | 181.7; 113.6–277.3 |
GBWR-Gain1 (%) | 0.69; 0.25–1.62 | 0.62; 0.25–1.19 | 0.76; 0.30–1.62 |
GBWR-Gain2 (%) | 0.94; 0.24–1.96 | 0.93; 0.58–1.52 | 0.96; 0.24–1.96 |
AT (cm3) | 6,975.5; 802–14,820 | 6,557; 1,973–14,820 | 7,229; 802–13,541 |
SAT (cm3) | 4,420; 112–12,386 | 4,604; 850–12,018 | 3,999; 112–12,386 |
VAT (cm3) | 1,844; 389–6,059 | 1,605; 389–4,828 | 2,747; 522–6,059 |
FMI (kg/m2) | 42.87; 4.14–98.1 | 316.5; 171–753 | 40.2; 4.1–81.8 |
sFMI (kg/m2) | 29.7; 0.58–80.63 | 46.2; 15.2–98.1 | 24.3; 0.58–61.4 |
vFMI (kg/m2) | 12.05; 2.17–44.26 | 33.6; 6.6–80.6 | 16.2; 2.2–44.3 |
Psoas (cm2) | 343; 114–753 | 11.3; 2.9–32.4 | 373; 114–698 |
Hospital-stay (d) | – | 12; 6–33 | – |
Mortality | – | 0 | – |
Morbidity | – | 16 | – |
Severe morbidity | – | 0 | – |
PT d3 (%) | – | 63; 38–89 | – |
Total bilirubin d3 (µmol/L) | – | 30; 8–140 | – |
PT to norm (d) | – | 4; 0–14 | – |
Bilirubin to norm (d) | – | 5; 0–37 | – |
LiMAx0 (µg/kg/h) | – | 441; 216–852 | – |
LiMAx1 (µg/kg/h) | – | 376; 108–730 | – |
LiMAx2 (µg/kg/h) | – | 421.5; 184–847 | – |
Data are shown as median; range. BMI, body mass index; TLV, total liver volume; FLR0, base line future liver remnant; interval1, between donation and early follow-up; interval2, between donation and late follow-up; FLR1, future liver remnant at the early follow-up; FLR2, future liver remnant at the late follow-up; Gain1, FLR-volume gain at the early follow-up; Gain2, FLR-volume gain at the late follow-up; GBWR, graft to body weight ratio; AT, estimated total adipose tissue in body stem; SAT, estimated subcutaneous adipose tissue in body stem; VAT, estimated visceral adipose tissue in body stem; FMI, fat mass index of body stem; sFMI, subcutaneous FMI; vFMI, visceral FMI. PMI, psoas mass index related to body stem, severe morbidity: > IIIa Clavien-Dindo; PT d3: Prothrombin time value at 3rd postoperative day, reference values: 70–130%; Total bilirubin d3: value at 3rd postoperative day, reference values: <21 µmol/L.
Liver volumetric assay and its correlation with fat analysis
The increase in liver volumes across the three time points (before surgery, the early and late liver volume measurements) was significant with the t-test as follows: FLR0vs. FLR1: P=5.7-33, FLR1vs. FLR2: P=0.000002 (Figure 1).
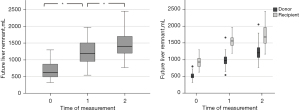
Initially, we analyzed the potential correlation between the fat mass index in the body stem and psoas mass from the one side and the percental liver volume gain from the other side. After that, we analyzed the variables, which show a linear correlation in a multiple regression. In the whole process, we performed a stratification into donor’s and recipient’s collective, since the percental liver volume gain in donors was significantly better than in recipients in early (P=0.0004) and late measurement (P=0.000005).
Early volumetric changes (after ca. 10 days)
Pearson’s correlation showed a significant correlation between the early percental liver volume gain from the one side and the following variables from the other side: age (r=−0.158, P=0.042), time interval between measurements (r=0.403, P=0.000002), sFMI (r=0.173, P=0.03) and Psoas mass (r=0.163, P=0.037). In a multiple regression containing the latter variables as independent variables and the early percental liver volume gain as a dependent variable only the interval (β =0.387, T =4.733, P=0.000006) and sFMI (β =0.396, T =2.464, P=0.015) remain showing a significant correlation. This means that extrahepatic adipose tissue could have a positive effect on the early liver volume gain after surgery.
In donors, Pearson’s correlation showed similar results to these in the main collective except the psoas mass (r=0.09, P=0.229). Multiple regression showed a significant correlation between sFMI and early percental liver volume gain (β =0.398, T =2.137, P=0.036).
In recipients, only time interval (r=0.261, P=0.033) and psoas mass showed a significant correlation with the volume gain. Multiple regression supported the correlation between psoas mass and volume gain in recipient’s collective (β =0.072, T =2.106, P=0.041). So that it could be said that the psoas could be a positive predictor for the liver volume gain after split transplantation.
Late volumetric changes (after ca. 6 months)
Regarding the late percental liver volume gain, only sFMI seemed to be significantly correlating with the liver volume gain (r=0.395, P=0.0004) (Figure 2).
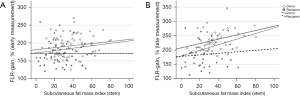
In stratification into donor’s and recipient’s collective, the correlation between sFMI and volume gain remain significant only in the donors (r=0.393, P=0.009). This supports again the supposition of positive effect of extrahepatic adipose tissue on liver volume gain in healthy donors after liver resection.
vFMI/sFMI
The impact of the ratio of vFMI/sFMI on FLR-gain was also analyzed. It shows a significant correlation between this ratio and early (r=−0.199, P=0.015) as well late (r=−0.276, P=0.006) FLR-gain. After stratification into donor’s and recipient’s subgroups, this effect stills significant only in donors and on late FLR-gain (r=−0.270, P=0.042).
Adjusted analysis regarding a narrower range of time of measurements
To overwhelm the matter of the wide range of the time between measurements we built subgroups regarding the percentiles. 94 (78.3%) early measurements were done after a time of 9 to 13 days. 61 (72.6%) late measurements were done after a time of 25 to 33 weeks. Accordingly, sFMI and vFMI/sFMI correlated significantly with early FLR-gain (r=0.234, P=0.012) and (r=−0.217, P=0.018) respectively and as well with late FLR-gain (r=0.375, P=0.001) and (r=−0.337, P=0.004) respectively. Regarding the same time ranges in donors, 53 (75.7%) early measurements were done after a time of 9 to 13 days. 33 (78.6%) late measurements were done after a time of 25 to 33 weeks. Accordingly, sFMI and vFMI/sFMI correlated significantly just with late FLR-gain (r=0.449, P=0.004) and (r=−0.343, P=0.025) respectively. There was no similar correlation in recipients.
Stratification according to gender
The ratio of vFMI/sFMI was compared according to gender. Mann-Whitney-U-test shows a significant difference of this ratio in the main collective (U =268, P=0.01-13), as in the donors (U =143, P=0.01-6) and recipients (U =21, P=0.0001) subgroup always with a higher vFMI/sFMI values in males. After stratification according to gender, only the correlation between sFMI and late FLR-gain in male donors was still significant (r=0.555, P=0.003), while no significant correlation was found in the recipients subgroup.
Normal weight vs. overweight/obesity state
Mann-Whitney-U-test shows no significant difference in early and late FLR-gain regarding weight state (P=0.296 and 0.062 respectively). In stratification into normal weight vs. overweight/obesity, only the correlation between sFMI and late FLR-gain was still significant with a weaker effect in the overweight/obesity group (r=0.333, P=0.014 and r=0.277, P=0.042 respectively). In donors and recipients subgroups, no significant correlation was found.
Liver function tests and their correlation with volumetric changes
There was no statistically significant relationship between LiMAx- and volumetric changes. Trends of bilirubin values also showed no statistically significant correlation with volumetric changes. Nevertheless, prothrombin time as a liver synthesis parameter showed a significant correlation to liver volume at the early measurement after surgery. The more liver volume on the early measurement [FLR1 (mL)] was, a higher PT value could be detected on the third postoperative day (r=0.271, P=0.023), and a lesser time PT value would take to return to reference range after surgery (r=−0.385, P=0.001). There was no direct correlation between LiMAx or laboratory trends and adipose tissue.
Early postoperative outcome
There was neither mortality nor severe morbidity (Clavien-Dindo > IIIa) (9,10) in our donor cohort. Sixteen donors had mild morbidity. The most common complication was a peripheral biliary leak, which was diagnosed in nine patients. No surgical revision was required in any of them. In five patients, endoscopic intervention via endoscopic retrograde cholangiography was performed to reduce the leak volume. The statistical correlation studies showed no relationship between adipose tissue and postoperative outcome regarding the development of complications and overall postoperative hospital stay.
Discussion
To our knowledge, this is the first clinical study describing the relationship between body fat tissue and liver regeneration. In this study, we investigated the positive impact of body adipose tissue on liver regeneration. We found that living donors with a higher fat mass index had a better liver volume gain after surgery. To the best of our knowledge, this is the first study in humans investigating the role of body adipose tissue in liver regeneration in otherwise healthy living liver donors. In addition to the physiological descriptions of transit fat accumulation in the regenerating liver (2-6,11), there are no clinical studies yet, so that this phenomenon cannot be confirmed in clinical practice.
Most commonly, liver regeneration is studied by performing a surgical procedure that removes 2/3 of the liver mass in rodents (rats and mice), a technique known as 2/3 partial hepatectomy (PHx) (12). Michalopoulos et al. have already described the mechanism of liver regeneration from biological and pathological aspects (11). He noticed a phenomenon of physiological accumulation of fatty droplets in hepatocytes during regeneration. We tried in our study to add a clinical aspect to this point of interest, which represents an essential aspect for the purpose of intact liver regeneration (3).
To avoid miscellaneous potential pathological factors that may affect liver regeneration, we decided to perform our analysis on living donors who underwent right hemihepatectomy. There was no mortality or only mild morbidity in the postoperative phase. The liver regeneration was not affected by severe complications. Therefore, we could explore the role of adipose tissue during liver regeneration in healthy individuals without disruptive risk factors that could impair the process of liver regeneration. This represents an advantage for isolated exploration of the role of adipose tissue.
We found a relationship between adipose tissue and liver regeneration capacity, which can support the previously mentioned description. Since it is supposed for liver regeneration to be impaired under immunosuppressive therapy, which was represented in our study in a significantly better regeneration in the donors collective, the analysis was made after stratification into donor’s and recipient’s subgroups. Therethrough, the impact of adipose tissue on liver regeneration was still significant only in the donor’s group. We noted a positive linear correlation between fat content in the body, especially the subcutaneous fat content, and liver regeneration capacity after right hemihepatectomy, while a similar correlation could not be detected when just considering BMI, height, or weight alone. Furthermore, the mass of the psoas muscle was irrelevant. Nevertheless, psoas represented a positive effect on liver regeneration only in the recipient’s subgroup. This goes along with the literature since there is an established negative effect of sarcopenia on the outcome after liver transplantation (13,14). The wide range of the time of early and late measurements of FLR-gain presents a limitation for this study, which could impair the study results, especially since the FLR-gain was significantly linearly correlated with the interval between the measurements. Therefore, the interval between measurements was included in the multivariate analysis. A better study design with strict time criteria for volumetric measurements should be undertaken in the future. Subcutaneous fat mass appeared to be significantly correlated with liver regeneration in our study, but with a weak to moderate correlation effect. From the correlation studies of vFMI/sFMI ratio, it refers to a negative impact of the visceral adipose tissue and support the positive impact of the subcutaneous adipose tissue on liver regeneration. However, this should not be taken as a clinical fact, since this could be due to the technical assessment of the adipose tissue by the software used in our study since this software detects the visceral fat depending on the Hounsfield unit threshold of the subcutaneous adipose tissue. Therethrough, it could come to better detection of subcutaneous adipose tissue. The distribution of adipose tissue differs according to gender in our collective. It shows a trend in visceral adipose distribution in males. After stratification according to gender, the correlation between FLR-gain and sFMI was only significantly found in male donors at the late liver volumetric measurement. Furthermore, it should be said, that the recognized regeneration preferability in our study based just on arithmetical aspect, which could not correlate absolutely to the functional aspect. This should be examined using methods, which offer an evaluation of liver function such as LiMAx test (8). Unfortunately, this test was performed sporadically on only a limited number of patients in our cohort, so we did not find a correlation between the LiMAx trends and liver volume changes. Another limitation of LiMAx lies in its implementation, which is postoperatively influenced by pain and temporary pleural effusion. Nevertheless, we found a relationship between Prothrombin time trends and liver volume changes, so we can say, that the latter does have an impact on liver function in the end.
There are certainly many other factors affecting liver regeneration that we do not yet know. For example, age seems to be one of these factors, as we showed in our analysis. The impairing role of age on liver regeneration was examined more than 50 years ago by Bucher et al. (15). At that time, Bucher et al. found that the regenerative response, although preserved, was considerably reduced and retarded in aged rodents after 2/3 partial hepatectomy. Several subsequent studies confirmed that observation (16-18). Stöcker and Heine showed that 99% of hepatocytes in the livers of young mice proliferate after PHx, whereas only 30% of hepatocytes in old livers undergo such proliferation (19). The interval between the two measurements is obviously another cofactor. Interestingly, these latter two factors would have no relevance when considering the late liver volume gain.
The relationship between adipose tissue and liver regeneration became more obvious when this was studied together with age and the time interval in a multiple regression analysis. Despite all of the factors mentioned above, this correlation was detected in a healthy cohort. Therefore, it is not applicable to all patients, especially patients with moderate to severe obesity, which is usually associated with fatty liver, and that situation actually impairs liver regeneration (2). In our cohort, the median BMI was 24.5 kg/m2, with a maximum of 34.1, which represents class 1 obesity. Certainly, people living with obesity would have a higher morbidity because of the correlated disorders. The patients with overweight/class 1 obesity in this study did not have an impairment of liver regeneration. The demographic design of this study represents a limitation, since there are no patients with obesity ≥ class 2, which is accompanied by a chronic inflammation and dysfunctional adipose tissue, which could impair the liver regeneration.
The clinical importance of the studied point lies in the fact that the adipose tissue in the body could represent a predictive factor for estimating the appropriateness of liver regeneration capacity preoperatively. This could help us to estimate the postresection regenerative capacity and to avoid significant morbidity and mortality after partial hepatectomy (1). Since the health of the living donor is the most important issue in living donor liver transplantation, this increases the safety of the donor even more. Furthermore, considering fat as a protective factor in liver regeneration, preconditioning of the patient before liver surgery through alimentary supplementation could improve liver regeneration after partial hepatectomy. The potential positive role of adipose tissue should be related to the adipokines, which regulate the metabolic adaptation of the liver to dietary fat. Alvarez-Sola et al. described the essential role of fibroblast growth factor 15/19 (FGF 15/19) on the regeneration in fatty liver (20). Other adipokines like Leptin as a regulator of food intake and body weight homeostasis and FGF19, which mainly related to body adiposity (21), should be also considered (22).
Based on the results of this current study, further prospective clinical trials with a robust design, strict criteria and considering the role of adipokines are needed to better understand and eventually utilize this protective effect in the interest of liver surgery and transplantation.
Conclusions
Subcutaneous adipose tissue could be a positive predictive factor to estimate the goodness of liver regeneration after partial hepatectomy. This could be helpful to improve the outcome after this type of surgery. Further prospective studies are necessary for better exploration of this research point of interest.
Acknowledgments
Funding: None.
Footnote
Reporting Checklist: The authors have completed the STROBE reporting checklist. Available at https://hbsn.amegroups.com/article/view/10.21037/hbsn-22-442/rc
Data Sharing Statement: Available at https://hbsn.amegroups.com/article/view/10.21037/hbsn-22-442/dss
Conflicts of Interest: All authors have completed the ICMJE uniform disclosure form (available at https://hbsn.amegroups.com/article/view/10.21037/hbsn-22-442/coif). The authors have no conflicts of interest to declare.
Ethical Statement: The authors are accountable for all aspects of the work in ensuring that questions related to the accuracy or integrity of any part of the work are appropriately investigated and resolved. The study was conducted in accordance with the Declaration of Helsinki (as revised in 2013). The study was initiated after approval by the local ethics committee of Friedrich-Schiller University, Jena (registration number 3826-07/13) and written informed consent was obtained from the individual patients.
Open Access Statement: This is an Open Access article distributed in accordance with the Creative Commons Attribution-NonCommercial-NoDerivs 4.0 International License (CC BY-NC-ND 4.0), which permits the non-commercial replication and distribution of the article with the strict proviso that no changes or edits are made and the original work is properly cited (including links to both the formal publication through the relevant DOI and the license). See: https://creativecommons.org/licenses/by-nc-nd/4.0/.
References
- van den Broek MA, Olde Damink SW, Dejong CH, et al. Liver failure after partial hepatic resection: definition, pathophysiology, risk factors and treatment. Liver Int 2008;28:767-80. [Crossref] [PubMed]
- Torbenson M, Yang SQ, Liu HZ, et al. STAT-3 overexpression and p21 up-regulation accompany impaired regeneration of fatty livers. Am J Pathol 2002;161:155-61. [Crossref] [PubMed]
- Shteyer E, Liao Y, Muglia LJ, et al. Disruption of hepatic adipogenesis is associated with impaired liver regeneration in mice. Hepatology 2004;40:1322-32. [Crossref] [PubMed]
- Michalopoulos GK, DeFrances MC. Liver regeneration. Science 1997;276:60-6. [Crossref] [PubMed]
- Delahunty TJ, Rubinstein D. Accumulation and release of triglycerides by rat liver following partial hepatectomy. J Lipid Res 1970;11:536-43. [Crossref] [PubMed]
- Yan XP, Wang S, Yang Y, et al. Effects of n-3 polyunsaturated fatty acids on rat livers after partial hepatectomy via LKB1-AMPK signaling pathway. Transplant Proc 2011;43:3604-12. [Crossref] [PubMed]
- Wolrd Medical Association. Declaration of Helsinki – Ethical principles for medical research involving human subjects. 64th WMA General Assembly, Fortaleza, Brazil, October 2013.
- Jara M, Dziodzio T, Malinowski M, et al. Prospective Assessment of Liver Function by an Enzymatic Liver Function Test to Estimate Short-Term Survival in Patients with Liver Cirrhosis. Dig Dis Sci 2019;64:576-84. [Crossref] [PubMed]
- Clavien PA, Sanabria JR, Strasberg SM. Proposed classification of complications of surgery with examples of utility in cholecystectomy. Surgery 1992;111:518-26. [PubMed]
- Dindo D, Demartines N, Clavien PA. Classification of surgical complications: a new proposal with evaluation in a cohort of 6336 patients and results of a survey. Ann Surg 2004;240:205-13. [Crossref] [PubMed]
- Michalopoulos GK. Liver regeneration. J Cell Physiol 2007;213:286-300. [Crossref] [PubMed]
- Higgins GM. Experimental pathology of the liver. I. Restoration of the liver of the white rat following partial surgical removal. Arch Pathol 1931;12:186-202.
- Freeman RB Jr, Wiesner RH, Harper A, et al. The new liver allocation system: moving toward evidence-based transplantation policy. Liver Transpl 2002;8:851-8. [Crossref] [PubMed]
- Merion RM, Wolfe RA, Dykstra DM, et al. Longitudinal assessment of mortality risk among candidates for liver transplantation. Liver Transpl 2003;9:12-8. [Crossref] [PubMed]
- Bucher NL, Swaffield MN, Ditroia JF. The influence of age upon the incorporation of thymidine-2-C14 into the DNA of regenerating rat liver. Cancer Res 1964;24:509-12. [PubMed]
- Fry M, Silber J, Loeb LA, et al. Delayed and reduced cell replication and diminishing levels of DNA polymerase-alpha in regenerating liver of aging mice. J Cell Physiol 1984;118:225-32. [Crossref] [PubMed]
- Iakova P, Awad SS, Timchenko NA. Aging reduces proliferative capacities of liver by switching pathways of C/EBPalpha growth arrest. Cell 2003;113:495-506. [Crossref] [PubMed]
- Timchenko NA. Aging and liver regeneration. Trends Endocrinol Metab 2009;20:171-6. [Crossref] [PubMed]
- Stöcker E, Heine WD. Regeneration of liver parenchyma under normal and pathological conditions. Beitr Pathol 1971;144:400-8. [PubMed]
- Alvarez-Sola G, Uriarte I, Latasa MU, et al. Fibroblast growth factor 15/19 (FGF15/19) protects from diet-induced hepatic steatosis: development of an FGF19-based chimeric molecule to promote fatty liver regeneration. Gut 2017;66:1818-28. [Crossref] [PubMed]
- Gómez-Ambrosi J, Gallego-Escuredo JM, Catalán V, et al. FGF19 and FGF21 serum concentrations in human obesity and type 2 diabetes behave differently after diet- or surgically-induced weight loss. Clin Nutr 2017;36:861-8. [Crossref] [PubMed]
- Muruzábal FJ, Frühbeck G, Gómez-Ambrosi J, et al. Immunocytochemical detection of leptin in non-mammalian vertebrate stomach. Gen Comp Endocrinol 2002;128:149-52. [Crossref] [PubMed]